Choroideremia
All content on Eyewiki is protected by copyright law and the Terms of Service. This content may not be reproduced, copied, or put into any artificial intelligence program, including large language and generative AI models, without permission from the Academy.
Disease Entity
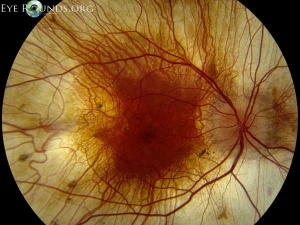
Choroideremia is recognized by the following codes as per the International Classification of Diseases (ICD) nomenclature:
ICD-9: 363.55
ICD-10: H31.21
OMIM Entry # 303100
Disease
Choroideremia is an X-linked chorioretinal dystrophy characterized by the diffuse, progressive degeneration of the retinal pigment epithelium (RPE), photoreceptors and choriocapillaris. It is caused by a mutation in the CHM gene and is the focus of exciting basic and clinical research. Gene therapy through viral vectors has shown early promise in the possible treatment of this blinding disease.
History
Choroideremia was first described in 1872 by Ludwig Mauthnuer, an Austrian ophthalmologist.[2] Initially, it was thought to be a developmental anomaly, similar to a choroidal coloboma, due to the near total lack of choroidal vessels.[3] Upon observation of less extreme cases, the progressive nature of the disease became apparent.[3] An X-linked connection was proposed in 1942, but it wasn’t until 1990 that the specific gene was cloned. [3][4] The CHM gene was one of the first genes to be identified by positional cloning and was one of the first genes to be established as a cause of an inherited retinal degeneration.[4][5][6][7] Since the early 1990s, over 106 pathogenic variations in the CHM gene have been discovered.[8] The most exciting developments have happened in the last several years, with the development of viral vectors designed to replace the mutated CHM gene.[9]
Definitions
Choroideremia derives its name from the almost complete loss of retina, choroid and RPE that leads to exposure of the underlying white sclera.[9] It is derived from “choroideremie”, which is thought to be a combination of the ancient Greek word “eremia,” meaning barren land or dessert, and “chorion”, which is ancient Greek for skin.[2]
Epidemiology
Choroideremia is a rare chorioretinal dystrophy that is estimated to affect between 1 in 50,000 to 1 in 100,000 individuals.[10] Men are predominantly affected due to its X-linked etiology, but women can be asymptomatic carriers or rarely can be affected by the dystrophy as well. Northern Finland has the highest reported prevalence.[11][12] There are thought to be more than 500 affected males in the United Kingdom and around 3000 throughout Europe.[13]
Genetics
Choroideremia is due to various mutations involving the CHM gene, which is located on chromosome Xq21.2, and is inherited in an X-linked recessive manner.[14] The gene spans 186,382 bp and the mRNA is made up of 15 exons and is 5442 bp long.[6] The open reading frame is 1,962 bp and produces a 653 amino acid long protein (95 kDa).[14] One hundred and six pathogenic variants in the CHM gene have been identified, and there are over 280 mutations that result in the CHM phenotype. [15][8] A variety of mutations have been discovered involving the CHM gene, including deletions, insertions, duplications, translocations, nonsense, splice-site, frameshift and missense mutations.[16] X-autosome translocations have been reported in female carriers presenting with mild clinical features of CHM in association with ovarian dysgenesis or with a more severe disease phenotype along with ectodermal dysplasia.[15]
Physiology and Pathology
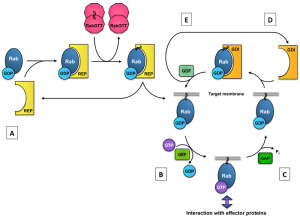
The CHM gene encodes for Rab escort protein-1 (REP-1). REP-1 is one of two Rab escort proteins, both of which are found throughout the body.[18] REP-1 is involved in a complex system of intracellular trafficking of various lipid membrane-bound structures. These vesicular structures are guided by GTP-binding proteins (Rab proteins).[10] For Rab proteins to be connected to the lipid membrane and allow intracellular trafficking, they need to be prenylated, which is the addition of geranylgeranyl groups to a molecule.[10][18][19] REP-1 proteins aid in this process by bringing Rab proteins to the Rab geranylgeranyltransferase (GGTase) complex, where the prenylation occurs.[18] REP-1 also facilitates the transfer of the prenylated Rab protein to its target location.[18] Without this escort, the prenylated Rab would undergo inactivation.[18] The figure to the left demonstrates the role of REP in the Rab cycle. [20]
Mutations in the CHM gene create defects in REP-1. This leads to improper intracellular vesicular trafficking and is thought to impair the transport of proteins from the Golgi apparatus to the outer segments in photoreceptors, as well as the impairment of phagocytosis and degradation of shed outer segments by RPE cells.[21]
Multiple types of mutations of the CHM gene have been described, but no particular type of mutation has been associated with a worse or better prognosis. This lack in phenotypic variability is not entirely surprising in that most mutations described show a near universal lack of REP-1 protein expression.[6][7][22] Variations in severity of disease must be due to factors other than the underlying mutation.
The specific anatomic location of the initial pathologic degeneration remains controversial. Some studies have suggested that the primary pathology in choroideremia resides in the RPE followed by degeneration of the photoreceptor layer and choroid.[23][24][25][26] Others have suggested the primary pathology develops in the photoreceptors, with subsequent loss of the RPE and choriocapillaris.[27][28][29] Finally, other examinations have suggested an independent loss of photoreceptors and RPE followed by degeneration of the choroid.[30][31] A recent histopathologic study found an inflammatory component to the disease process along with prominent gliosis, with gliosis likely being a secondary event.[13]
Diagnosis
Symptoms
Choroideremia becomes symptomatic during the first decade of childhood with the development of nyctalopia. It then progresses to peripheral vision loss in teenage years with sparing of central vision and maintenance of good visual acuity until the fifth to seventh decade of life.[10][32] At around the fifth decade of life, most patients develop a rapid deterioration in central vision.[33] Color vision is lost as degeneration of the macula takes place, and can occur prior to loss of visual acuity.[32][34] Phenotypic variation within a family can be striking, with some affected males significantly less affected than other males.[35] Carriers are generally asymptomatic, though female carriers may present with mild to moderate nyctalopia, with clinical findings of chorioretinopathy, RPE atrophy and granular pigmentary atrophy in the periphery.[36][37]
Fundus Examination
On fundus examination, the earliest manifestation is widespread pigment clumping at the level of the RPE, which is distinct from the characteristic perivascular bone-spicule pigment clumping seen in retinitis pigmentosa.[10] Subsequently, patients develop well-defined regions of atrophy with visible underlying sclera and large choroidal vessels, most commonly in the postequatorial region just outside the vascular arcades. These areas of atrophy advance centripetally and are also found in a peripapillary and parapapillary manner (See image below).[10] An island of foveal tissue may persist until later stages of the disease when central and color vision become affected from foveal atrophy. Patients have preserved larger choroidal blood vessels and normal appearing retinal vessels. In addition, choroideremia exhibits no optic atrophy, unlike the waxy pallor of optic discs seen in retinitis pigmentosa. Carrier patients can have mild RPE changes, and in severe cases, patchy RPE degeneration and areas of atrophy.[10][38] This phenotypic variability in carriers is due to lyonization in which one copy of the X chromosome is randomly silenced early in embryogenesis. Other associated ocular findings include 31% of patients developing posterior subcapsular cataracts and a small risk of macular edema or choroidal neovascularization.[39]
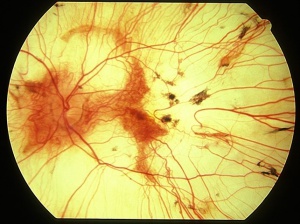
Fluorescein angiography
On fluorescein angiography, scalloped areas of missing choriocapillaris appear hypofluorescent next to brightly hyperfluorescent areas of perfused choriocapillaris (See image below). Although not a common feature, choroidal neovascularization secondary to choroideremia can develop, leading to leakage characteristic of neovascularization.[41][42][43][44][45]
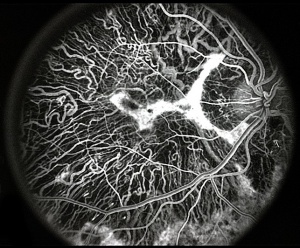
Fundus Autofluorescence
Fundus autofluorescence can show early loss of peripheral autofluorescence with subsequent centripetal loss.[47] The scalloped edges of demarcation are sharp and can clarify areas of the fundus that are affected and not apparent on fundus examination (See images below). Hypoautofluorescence in the nonatrophic areas may precede photoreceptor cell death, and the amount of hypoautofluorescence may help predict disease activity and help identify patients undergoing a more rapid degeneration. A speckled autofluorescence may be observed in an otherwise unaffected macula in both affected males and carriers.[10][48] Nasal retinal sensitivity appeared to decline earlier than temporal retina, which mirrors a similar pattern of FAF island shrinkage.[49]
![]() This fundus autofluorescent image is of a female choroideremia carrrier. The lyonization found with this disease can be seen with the patchy distribution of atrophic areas.[50] |
![]() This figure is a fundus photograph (A) and the associated fundus autofluorescence (C) of a patient with choroideremia. Note the better characterization of diseased and healthy retina on fundus autofluorescence that is not as apparent as on the fundus photograph. Image B was performed in retromode and image D is an NIR-AF image.[51] |
Electroretinography
Electroretinography is abnormal early in the course of the disease, with a reduced scotopic component before the photopic component.[52][53][54] It becomes extinguished by midlife.[54] Carriers generally have a normal ERG pattern but symptomatic carriers may develop minor changes, in particular a subnormal 30-Hz flicker response on full-field ERG or diffuse elevation of 650-nm dark-adapted thresholds.[25][38][53]
Optical Coherence Tomography
Optical coherence tomography demonstrates preservation of the inner retinal layers throughout the disease process.[10] Patients tend to have a small increase in central retinal thickness early in the course of disease when normal visual acuity is present, but eventually develop progressive subfoveal retinal thinning as visual acuity declines.[10][32] OCT can demonstrate a reduction in subfoveal choroidal thickness. As the disease progresses, patients often develop retinal tubulations in the outer nuclear layers and inner retinal microcysts. [21][31][32] One study showed that 62.5 % of patients showed some degree of cystoid macular edema on OCT.[55]
Visual Field
Visual field loss is consistent with the location of chorioretinal degeneration. The earliest field change is a patchy loss of midperipheral vision.[32] Progressive, patchy atrophy can lead to irregular scotomas and eventually to near complete loss of a central and peripheral vision.[32] In advanced disease small islands of vision can remain in the fovea and light can be perceived in the far periphery.[56]
OCT Angiography
OCT angiography is a noninvasive, functional extension of OCT for visualizing retinal and choroidal microvasculature. Advanced cases of choroideremia can result in the distortion of the neurosensory retina, RPE and choriocapillaris, which can complicate the interpretation of both FA and OCT. Patel et al demonstrated the use of OCT angiography in the characterization and quantification of CNV in a patient with choroideremia.[57] OCTA demonstrated severe changes in the deep capillary plexus in both the central preserved retinal islet and the surrounding atrophic area and alterations in the choriocapillaris in the atrophic area.[58]Abbouda et al demonstrated that the superficial retinal vascular network had a significantly lower area of capillary plexus with flow in affected males compared with female carriers and controls.[59] More research is necessary to clarify the role of this new technology in the management of choroideremia.
Differential diagnosis with defining features
- Gyrate atrophy: Choroideremia in early stages may mimic gyrate atrophy of retina and choroid. Examination of fundus of family members, early presentation, and X-linked inheritance pattern are important features to clinically differentiate choroideremia from gyrate atrophy. Autosomal recessive, well demarcated scalloped areas of chorioretinal atrophy, nyctalopia in second to third decade, systemic hyperornithinemia, myopia and early cataracts[60]
- Retinitis Pigmentosa: Waxy disc pallor, peripheral RPE bone spicule like degeneration, retinal arteriolar attenuation[61]
- Myopic Degeneration: Absence of nyctalopia, tessellated fundus, lacquer cracks, diffuse atrophy, patchy atrophy, posterior staphyloma, high axial length, macular atrophy, straightened and stretched vessels, high rates of choroidal neovascularization, temporal peripapillary atrophic crescent, hemorrhages and tilting of the optic disc[62]
- Ocular albinism: Infantile nystagmus, iris translucency, substantial hypopigmentation of ocular fundus, foveal hypoplasia, aberrant optic pathway projection associated with asymmetry of cortical responses on visual evoked potential testing[63]
- Usher syndrome type 1: Autosomal recessive, pigmentary retinopathy, congenital deafness, imbalance from vestibular dysfunction[64]
- Thioridazine hydrochloride retinal toxicity: History of medication use, loss of night vision, decreased ERG amplitudes, accumulation of fine or coarse pigment clumps, geographic RPE and choriocapillary atrophy[65]
- Bietti crystalline dystrophy: Autosomal recessive, corneal deposits, yellow-white crystalline retinal deposits, progressive atrophy of the RPE, loss of choriocapillaris, progressive nyctalopia, visual field constriction, legal blindness in the fifth or sixth decades of life[66]
![]() This is a fundus photograph of a patient with gyrate atrophy. Note the hyperpigmentation of the remaining RPE, in comparison to choroideremia.[67] |
![]() This is a fundus photograph of a patient with retinitis pigmentosa. Note the bone-spicule pigment clumping and optic nerve pallor that differentiates this disease from choroideremia.[68] |
![]() This image is a fundus photograph of a patient with myopic degeneration. Note involvement of the center of the macula, which is affected sooner in myopic degeneration than in choroideremia.[69] |
![]() This is a fundus image of a patient with ocular albinism. Note the diffuse absence of pigmentation, in comparison to the scalloped, patchy loss of pigmentation in choroideremia.[70] |
Laboratory tests
The diagnosis of choroideremia can be suggested by characteristic fundus findings and family history. It can be confirmed by direct genetic testing or through immunoblot analysis with anti-REP-1 antibody.[71] Confirmation is warranted due to phenotypic variation and clinical overlap with other conditions, with possible therapeutic and prognostic relevance if an alternative diagnosis is made.[72] Prenatal testing for choroideremia has been described using next-generation sequencing in a Chinese cohort using cell free fetal DNA.[73]
Management
Investigational Therapies
Gene delivery through the Adeno-Associated Virus Subtype 2 Viral Vector
Basics
Recent success in the treatment of Leber’s congenital amaurosis has brought hope to developing a successful therapy for choroideremia.[74][75][76] Since choroideremia is a genetic disease and caused by a mutation of one gene, it is a promising candidate for successful gene therapy. [77] Recent investigational therapies have focused on replacing the defective gene via viral vectors. Adeno-associated virus subtype 2 (AAV2) has been of particular interest in ophthalmic research due to its affinity for primate photoreceptors and RPE.[78] This subtype of adeno-associated viruses also has the advantage of being well characterized in a number of animal models.[78] The amount of genetic material that can be transmitted by a virus is limited by the packaging capacity of the vector, so the size of the gene to be replaced is important. The limit of AAV vectors is estimated to around 5kb for single stranded DNA, which is much greater than the approximate 1.9kb coding sequence of REP-1.[79][80] Prior to the investigational treatment in humans, the replacement of the REP-1 gene was first investigated in mice. REP-1 replacement via the AAV2 vector was demonstrated to be feasible by Tamolchova et al.[78] In addition, they demonstrated an improvement in ERG responses in mice following subretinal injections of the designed viral vector.[78] These studies are promising because they show the feasibility and tolerability of gene therapy through a viral vector in humans with choroideremia. More studies will be needed to investigate further clarify the effectiveness and safety of this therapy.
Recent Studies
In a recent, multicenter phase 1/2 clinical trial, six male patients were administered with subretinally placed AAV.REP1.[9] The first step in the surgical procedure was to detach the target retina with the use of balanced salt solution injected through a 41G Teflon cannula. Once detached, a fixed volume (0.1mL) of 1×1010 AAV2.REP1 genome particles was injected into the subretinal space in 5 of 6 patients. In the sixth patient, a dose of 6×109 was injected. The injection of viral particles resulted in extension of the detachment in all patients. The sixth patient was injected with a smaller dose of viral particles because of difficulty in creating a detachment in that patient and concern about stretching the papillomacular bundle. At six months, the mean change in ETDRS letters was +3.8 letters in the treated eyes in comparison to +1.5 letters in the control eyes. Statistical analysis was not published in this study due to small sample size. Patient 1 had a +21 letter improvement and patient 4 had a +11 letter improvement, with the other patients have a marginal loss in visual acuity. On microperimetry, a mean increase was noted in retinal sensitivity with respect to the dimmest stimulus seen, mean retinal sensitivity and total number of test points seen, in contrast to a reduction in all of these parameters in the patient's contralateral eye. In regards to safety, there was no observed immune response to the injected viral particles. Two patients had mild distortion of central vision which resolved by 6 months. All patients had sub-clinical progression of lens opacification, as expected following vitrectomy. No serious systemic or ocular adverse events were reported.[9] At 3.5 years, the patients who had initial improvement in visual acuity maintained that visual acuity. [81]Three other patients did not have a significant change in visual acuity and one patient had a decline in vision. [81]The patient who had a decline in vision was the patient injected with a lower total vector dose.[81]
Xue et al utilized an adenoviral-associated viral vector to express REP1 and was delivered via subretinal injection in one eye of 14 patients with choroideremia. Five patients were treated with low dose of 1x 1010 genome particles and the remaining were treated with a high dose of 1x1011 genome particles. Oral prednisolone was administered orally following subretinal injection for immunosuppression. Two patients were noted to have complications, which included a surgical complication that results in retinal thinning and retinal inflammation noted 2 weeks post-operatively. Microperimetry was utilized to assess retinal sensitivity with no significant difference noted between the two groups. After two years, BCVA improved with a mean 4.5 letter gain and 6 treated eyes gaining more than one line of vision.[82]
Lam et al. evaluated the safety and efficacy of high-dose gene therapy in patients with choroideremia in a 2 year clinical trial. Six men with advanced choroideremia underwent subfoveal injection of adeno-associated virus 2 capsids that harbored a transcript encoding Rab escort protein 1. The eye with worse visual acuity was treated, and the untreated fellow eye served as the control. The mode of delivery was slow injection with microscope integrated intra-operative OCT. The primary outcome measure was change in BCVA from baseline. Secondary endpoints included changes in central visual field by microperimetry, color vision, contrast sensitivity, and fundus autofluorescence. The baseline mean BCVA was 65.3 ± 8.8 letters in treated eyes and 77.0 ± 4.2 letters in untreated eyes. Two years after therapy, the changes from baseline ranged from −1 to +10 letters in treated eyes and −2 to +4 letters in untreated eyes. There were no significant changes in the secondary endpoints assessed, though all eyes had progressive shrinkage in areas of fundus autofluorescence. Two patients were noted to have an atrophic retinal hole developed in a nonfunctioning macular area.
In a phase 1/2 clinical trial, Maclaren et al evaluated six male patients with a clinical phenotype of choroideremia who underwent subfoveal injection of AAV.REP1 (0·6–1·0×1010 genome particles). The patients were selected to represent different stages of the disease, including a normal foveal structure, partial foveal collapse, and complete foveal loss. Outcome measures included BCVA, microperimetry, and retinal sensitivity tests. Of the 6 patients, 2 patients gained +21 and +11 letters, while 4 patients with near normal best corrected visual acuity at baseline recovered to within one to three letters. Over 6 months, the increase in retinal sensitivity in the treated eyes correlated with the vector dose administered per mm2 of surviving retina in all eyes. Interestingly, one patient in whom the vector was not administered to the fovea re-established variable eccentric fixation that included the ectopic island of remaining RPE that had been exposed to vector.[83]
Challenges of Gene Therapy
With the era of subretinal gene therapy on the horizon, there are noted challenges with regards to patient selection and surgical technique. In addition to confirmation of the gene mutation, surgeons can also include an assessment of retinal structure and selection of a target zone for treatment. The anatomical features of disease progression are relevant to gene therapy approaches because the volume of tissue to be targeted is continually shrinking and the multiplicity of infection of viral particles per cell will vary at different stages of the disease. Other challenges include an unpredictable expansion of the bleb area, variable response of the retinal tissue and variable adhesion in different IRDs, and the challenge of avoided excess retinal stretch and reflux of the vector into the vitreous. At the time of this article, little has been published on techniques for subretinal gene injection and outcomes.[84][85]
Current Viral Vector Studies
Here is a global list of clinical trials into the treatment of choroideremia through viral vectors:
Future Directions
Retinal prosthesis devices have been implanted in patients with IRDs. The data from the one year safety and performance assessment of the Argus II (Second Sight Medical Prducts, Sylmar, California, USA) system described implantation in 47 patients, one of which had choroideremia. This consists of an epiretinal stimulating array that is implanted in the retina and transmits signals to the visual cortex.[86] Another approach to increase expression of RPE1 is to utilized small-molecule drugs that can promote ribosomal read-through of premature stop codons and bypass abnormal termination signals. In a nonsense-mediated zebrafish model of choroideremia , use of these small-moecule drugs lead to an increase in REP1 protein. [87]
Lutein
Lutein has been investigated as a supplement to reduce the progression of atrophy and vision loss in choroideremia. Lutein is a carotenoid that is present in high concentrations in the macula. It works as a filter protecting the macula from blue light and functions as a free radical scavenger and antioxidant.[88] Supplementation with oral lutein (20 mg per day) has been investigated over a 6 month period. [89]Results demonstrated an increase in serum lutein and macular pigment levels, but absolute foveal sensitivity did not change, suggesting no short term benefit from supplementation.[89] No studies looking into the long-term effectiveness of oral lutein supplementation have been conducted.[89]
Treatment of Macular Edema
Frank macular edema is not a typical feature of choroideremia, but its development may result in a sudden decline in central visual acuity. The pathogenic etiology of macular edema in patients with retinal dystrophies remains poorly understood. It may be treated with topical dorzolamide, which was demonstrated to be effective in a small study.[90] This therapy is similar to the management of macular edema found in other retinal dystrophies such as retinitis pigmentosa.[91][92] While the edema may be responsive to anti-VEGF agents as demonstrated in retinitis pigmentosa patients, it may be more appropriate to begin with topical carbonic anhydrase inhibitor therapy and if that fails then move to more invasive options.[93][94]
Considerations for Cataract Surgery in Choroideremia Patients
Surgeons may be appropriately concerned about performing cataract surgery in patients with choroideremia. Patients are placed at risk of phototoxicity from microscope illumination as well as at risk of postoperative pseudophakic macular edema. Few studies have been performed looking at outcomes of cataract surgery in this patient population. One study, a case series of 6 patients, demonstrated an improvement in visual acuity with cataract surgery without the development of postoperative macular edema.[95] Two patients did develop early capsular phimosis however, suggesting this may be a feature of the disease. [95] When performing cataract surgery in patients with choroideremia, it is important to obtain appropriate informed consent and educating patients about the potential risks of the procedure and the unknowns of the post-operative course.
References
- ↑ Welder D, Jeffrey D. Photographer: Toni Venckus. Choroideremia. © of EyeRounds.org of The University of Iowa. Permissions obtained from executive director and editor of Eyerounds.org.This image was originally published in the Ophthalmic Atlas Images by EyeRounds.org of The University of Iowa.This image is licensed under the Creative Commons Attribution-NonCommercial-NoDerivs 3.0 Unported License.
- ↑ 2.0 2.1 Barnard AR, Groppe M, MacLaren RE. Gene therapy for choroideremia using an adeno-associated viral (AAV) vector. Cold Spring Harbor perspectives in medicine. 2014;5(3):a017293.
- ↑ 3.0 3.1 3.2 Sorsby A, Franceschetti A, Joseph R, Davey JB. Choroideremia; clinical and genetic aspects. The British journal of ophthalmology. 1952;36(10):547-581.
- ↑ 4.0 4.1 Cremers FP, Brunsmann F, Berger W, et al. Cloning of the breakpoints of a deletion associated with choroidermia. Human genetics. 1990;86(1):61-64.
- ↑ van Bokhoven H, van den Hurk JA, Bogerd L, et al. Cloning and characterization of the human choroideremia gene. Human molecular genetics. 1994;3(7):1041-1046.
- ↑ 6.0 6.1 6.2 Huckfeldt RM, Bennett J. Promising first steps in gene therapy for choroideremia. Human gene therapy. 2014;25(2):96-97.
- ↑ 7.0 7.1 Seabra MC, Brown MS, Goldstein JL. Retinal degeneration in choroideremia: deficiency of rab geranylgeranyl transferase. Science (New York, N.Y.). 1993;259(5093):377-381.
- ↑ 8.0 8.1 Freund PR, Sergeev YV, MacDonald IM. Analysis of a large choroideremia dataset does not suggest a preference for inclusion of certain genotypes in future trials of gene therapy. Molecular genetics & genomic medicine. 2016;4(3):344-358.
- ↑ 9.0 9.1 9.2 9.3 MacLaren RE, Groppe M, Barnard AR, et al. Retinal gene therapy in patients with choroideremia: initial findings from a phase 1/2 clinical trial. Lancet (London, England). 2014;383(9923):1129-1137.
- ↑ 10.0 10.1 10.2 10.3 10.4 10.5 10.6 10.7 10.8 10.9 Khan KN, Islam F, Moore AT, Michaelides M. Clinical and Genetic Features of Choroideremia in Childhood. Ophthalmology. 2016;123(10):2158-2165.
- ↑ Sankila EM, Tolvanen R, van den Hurk JA, Cremers FP, de la Chapelle A. Aberrant splicing of the CHM gene is a significant cause of choroideremia. Nature genetics. 1992;1(2):109-113.
- ↑ Karna J. Choroideremia. A clinical and genetic study of 84 Finnish patients and 126 female carriers. Acta ophthalmologica. Supplement. 1986;176:1-68.
- ↑ 13.0 13.1 MacDonald IM, Russell L, Chan CC. Choroideremia: new findings from ocular pathology and review of recent literature. Survey of ophthalmology. 2009;54(3):401-407.
- ↑ 14.0 14.1 Furgoch MJ, Mewes-Ares J, Radziwon A, Macdonald IM. Molecular genetic diagnostic techniques in choroideremia. Molecular vision. 2014;20:535-544.
- ↑ 15.0 15.1 Mitsios, Andreas, Adam M. Dubis, and Mariya Moosajee. "Choroideremia: from genetic and clinical phenotyping to gene therapy and future treatments." Therapeutic advances in ophthalmology 10 (2018): 2515841418817490.
- ↑ Sanchez-Alcudia R, Garcia-Hoyos M, Lopez-Martinez MA, et al. A Comprehensive Analysis of Choroideremia: From Genetic Characterization to Clinical Practice. PloS one. 2016;11(4):e0151943.
- ↑ Kotzcsh M, Goettig P, Soelch S, Magdolen V. RAB31 (Ras-related protein in brain 31); Atlas Genet Cytogenet Oncol Haematol. In press. On line version: http://atlasgeneticsoncology.org/Genes/RAB31ID41978ch18p11.html. Permission to use image obtained from both corresponding author and journal. © Atlas of Genetics and Cytogenetics in Oncology and Haematology.
- ↑ 18.0 18.1 18.2 18.3 18.4 Preising M, Ayuso C. Rab escort protein 1 (REP1) in intracellular traffic: a functional and pathophysiological overview. Ophthalmic genetics. 2004;25(2):101-110.
- ↑ Seabra MC, Brown MS, Slaughter CA, Sudhof TC, Goldstein JL. Purification of component A of Rab geranylgeranyl transferase: possible identity with the choroideremia gene product. Cell. 1992;70(6):1049-1057.
- ↑ Kotzcsh M, Goettig P, Soelch S, Magdolen V. RAB31 (Ras-related protein in brain 31); Atlas Genet Cytogenet Oncol Haematol. In press. On line version: http://atlasgeneticsoncology.org/Genes/RAB31ID41978ch18p11.html
- ↑ 21.0 21.1 Xue K, Oldani M, Jolly JK, et al. Correlation of Optical Coherence Tomography and Autofluorescence in the Outer Retina and Choroid of Patients With Choroideremia. Investigative ophthalmology & visual science. 2016;57(8):3674-3684.
- ↑ Simunovic MP, Jolly JK, Xue K, et al. The Spectrum of CHM Gene Mutations in Choroideremia and Their Relationship to Clinical Phenotype. Investigative ophthalmology & visual science. 2016;57(14):6033-6039.
- ↑ Flannery JG, Bird AC, Farber DB, Weleber RG, Bok D. A histopathologic study of a choroideremia carrier. Investigative ophthalmology & visual science. 1990;31(2):229-236.
- ↑ Morgan JI, Han G, Klinman E, et al. High-resolution adaptive optics retinal imaging of cellular structure in choroideremia. Investigative ophthalmology & visual science. 2014;55(10):6381-6397.
- ↑ 25.0 25.1 Mura M, Sereda C, Jablonski MM, MacDonald IM, Iannaccone A. Clinical and functional findings in choroideremia due to complete deletion of the CHM gene. Archives of ophthalmology (Chicago, Ill. : 1960). 2007;125(8):1107-1113.
- ↑ Jain N, Jia Y, Gao SS, et al. Optical Coherence Tomography Angiography in Choroideremia: Correlating Choriocapillaris Loss With Overlying Degeneration. JAMA ophthalmology. 2016;134(6):697-702.
- ↑ Syed N, Smith JE, John SK, Seabra MC, Aguirre GD, Milam AH. Evaluation of retinal photoreceptors and pigment epithelium in a female carrier of choroideremia. Ophthalmology. 2001;108(4):711-720.
- ↑ Jacobson SG, Cideciyan AV, Sumaroka A, et al. Remodeling of the human retina in choroideremia: rab escort protein 1 (REP-1) mutations. Investigative ophthalmology & visual science. 2006;47(9):4113-4120.
- ↑ Lazow MA, Hood DC, Ramachandran R, et al. Transition zones between healthy and diseased retina in choroideremia (CHM) and Stargardt disease (STGD) as compared to retinitis pigmentosa (RP). Investigative ophthalmology & visual science. 2011;52(13):9581-9590.
- ↑ Bonilha VL, Trzupek KM, Li Y, et al. Choroideremia: analysis of the retina from a female symptomatic carrier. Ophthalmic genetics. 2008;29(3):99-110.
- ↑ 31.0 31.1 Syed R, Sundquist SM, Ratnam K, et al. High-resolution images of retinal structure in patients with choroideremia. Investigative ophthalmology & visual science. 2013;54(2):950-961.
- ↑ 32.0 32.1 32.2 32.3 32.4 32.5 Heon E, Alabduljalil T, Iii DB, et al. Visual Function and Central Retinal Structure in Choroideremia. Investigative ophthalmology & visual science. 2016;57(9):Oct377-387.
- ↑ Coussa RG, Kim J, Traboulsi EI. Choroideremia: effect of age on visual acuity in patients and female carriers. Ophthalmic genetics. 2012;33(2):66-73.
- ↑ Jolly JK, Groppe M, Birks J, Downes SM, MacLaren RE. Functional Defects in Color Vision in Patients With Choroideremia. American journal of ophthalmology. 2015;160(4):822-831.e823.
- ↑ Ponjavic V, Abrahamson M, Andreasson S, et al. Phenotype variations within a choroideremia family lacking the entire CHM gene. Ophthalmic genetics. 1995;16(4):143-150.
- ↑ Dugel PU, Zimmer CN, Shahidi AM. A case study of choroideremia carrier - Use of multi-spectral imaging in highlighting clinical features. Am J Ophthalmol Case Rep. 2016;2:18-22. Published 2016 Apr 14. doi:10.1016/j.ajoc.2016.04.003
- ↑ Jauregui, Ruben, et al. "Spectrum of disease severity and phenotype in choroideremia carriers." American journal of ophthalmology 207 (2019): 77-86.
- ↑ 38.0 38.1 Renner AB, Fiebig BS, Cropp E, Weber BH, Kellner U. Progression of retinal pigment epithelial alterations during long-term follow-up in female carriers of choroideremia and report of a novel CHM mutation. Archives of ophthalmology (Chicago, Ill. : 1960). 2009;127(7):907-912.
- ↑ MacDonald IM, Hume S, Chan S, Seabra MC. Choroideremia. In: Pagon RA, Adam MP, Ardinger HH, et al., eds. GeneReviews(R). Seattle (WA): University of Washington, Seattle University of Washington, Seattle. All rights reserved.; 1993.
- ↑ Kaplan JH, Piri N. Choroideremia. Retina Image Bank. March 29, 2013; Image Number 5373. © The American Society of Retina Specialists. Permission for use obtained from Director of Retina Image Bank and Members Section of the ASRS. This image was originally published in the Retina Image Bank.
- ↑ Campos-Pavon J, Torres-Pena JL. Choroidal neovascularization secondary to choroideremia. Archivos de la Sociedad Espanola de Oftalmologia. 2015;90(6):289-291.
- ↑ Sawa M, Tamaki Y, Klancnik JM, Jr., Yannuzzi LA. Intraretinal foveal neovascularization in choroideremia. Retina (Philadelphia, Pa.). 2006;26(5):585-588.
- ↑ Endo K, Yuzawa M, Ohba N. Choroideremia associated with subretinal neovascular membrane. Acta ophthalmologica Scandinavica. 2000;78(4):483-486.
- ↑ Robinson D, Tiedeman J. Choroideremia associated with a subretinal neovascular membrane. Case report. Retina (Philadelphia, Pa.). 1987;7(2):70-74.
- ↑ Sanchez-Alcudia R, Garcia-Hoyos M, Lopez-Martinez MA, et al. A Comprehensive Analysis of Choroideremia: From Genetic Characterization to Clinical Practice. PloS one. 2016;11(4):e0151943.
- ↑ Kaplan HJ, Piri N. Choroideremia. Retina Image Bank. March 29, 2013; Image Number 5375. © The American Society of Retina Specialists.Permission for use obtained from Director of Retina Image Bank and Members Section of the ASRS. This image was originally published in the Retina Image Bank.
- ↑ Jolly JK, Edwards TL, Moules J, Groppe M, Downes SM, MacLaren RE. A Qualitative and Quantitative Assessment of Fundus Autofluorescence Patterns in Patients With Choroideremia. Investigative ophthalmology & visual science. 2016;57(10):4498-4503.
- ↑ Preising MN, Wegscheider E, Friedburg C, Poloschek CM, Wabbels BK, Lorenz B. Fundus autofluorescence in carriers of choroideremia and correlation with electrophysiologic and psychophysical data. Ophthalmology. 2009;116(6):1201-1209.e1201-1202.
- ↑ Jolly, J. K., Edwards, T. L., Moules, J., Groppe, M., Downes, S. M., & MacLaren, R. E. (2016). A qualitative and quantitative assessment of fundus autofluorescence patterns in patients with choroideremia. Investigative ophthalmology & visual science, 57(10), 4498-4503.
- ↑ This image was originally published in the Retina Gallery. No author information was provided on the site. Permission for use obtained from Retina Gallery webmaster. File 12/37.
- ↑ Maurizio BP, Pierluigi I, Stelios K, Stefano V, Marialucia C, Ilaria Z, Francesco B. Retro-mode imaging and fundus autofluorescence with scanning laser ophthalmoscope of retinal dystrophies. This image is allowed to be used if properly cited, as per the Creative Commons Attribution License.© Parodi et al.; licensee BioMed Central Ltd. 2012.This image was originally published by BMC Ophthalmology.
- ↑ Zhou Q, Liu L, Xu F, et al. Genetic and phenotypic characteristics of three Mainland Chinese families with choroideremia. Molecular vision. 2012;18:309-316.
- ↑ 53.0 53.1 Sieving PA, Niffenegger JH, Berson EL. Electroretinographic findings in selected pedigrees with choroideremia. American journal of ophthalmology. 1986;101(3):361-367.
- ↑ 54.0 54.1 Renner AB, Kellner U, Cropp E, et al. Choroideremia: variability of clinical and electrophysiological characteristics and first report of a negative electroretinogram. Ophthalmology. 2006;113(11):2066.e2061-2010.
- ↑ Genead MA, Fishman GA. Cystic macular oedema on spectral-domain optical coherence tomography in choroideremia patients without cystic changes on fundus examination. Eye (London, England). 2011;25(1):84-90.
- ↑ McCulloch C. Choroideremia: a clinical and pathologic review. Transactions of the American Ophthalmological Society. 1969;67:142-195.
- ↑ Patel RC, Gao SS, Zhang M, et al. OPTICAL COHERENCE TOMOGRAPHY ANGIOGRAPHY OF CHOROIDAL NEOVASCULARIZATION IN FOUR INHERITED RETINAL DYSTROPHIES. Retina (Philadelphia, Pa). 2016;36(12):2339-2347.
- ↑ Source: Arrigo, Alessandro, et al. "Reduced vessel density in deep capillary plexus correlates with retinal layer thickness in choroideremia." British Journal of Ophthalmology 105.5 (2021): 687-693.
- ↑ Abbouda, A., et al. "Identifying characteristic features of the retinal and choroidal vasculature in choroideremia using optical coherence tomography angiography." Eye 32.3 (2018): 563-571.
- ↑ Sergouniotis PI, Davidson AE, Lenassi E, Devery SR, Moore AT, Webster AR. Retinal structure, function, and molecular pathologic features in gyrate atrophy. Ophthalmology. 2012;119(3):596-605.
- ↑ Hartong DT, Berson EL, Dryja TP. Retinitis pigmentosa. Lancet (London, England). 2006;368(9549):1795-1809.
- ↑ Silva R. Myopic maculopathy: a review. Ophthalmologica. Journal international d'ophtalmologie. International journal of ophthalmology. Zeitschrift fur Augenheilkunde. 2012;228(4):197-213.
- ↑ Lewis RA. Ocular Albinism, X-Linked. In: Pagon RA, Adam MP, Ardinger HH, et al., eds.GeneReviews(R). Seattle (WA): University of Washington, Seattle University of Washington, Seattle. All rights reserved.; 1993.
- ↑ Eudy JD, Sumegi J. Molecular genetics of Usher syndrome. Cellular and molecular life sciences : CMLS. 1999;56(3-4):258-267.
- ↑ Borodoker N, Del Priore LV, De ACC, Yannuzzi LA. Retinopathy as a result of long-term use of thioridazine. Archives of ophthalmology (Chicago, Ill. : 1960). 2002;120(7):994-995.
- ↑ Halford S, Liew G, Mackay DS, et al. Detailed phenotypic and genotypic characterization of bietti crystalline dystrophy. Ophthalmology. 2014;121(6):1174-1184.
- ↑ Vislisel, J. Gyrate Atrophy. This image was originally published in the Ophthalmic Atlas Images by EyeRounds.org of The University of Iowa. This image is licensed under the [/eyewiki.org/Creative_Commons_Attribution-NonCommercial-NoDerivs_3.0_Unported_License Creative Commons Attribution-NonCommercial-NoDerivs 3.0 Unported License]. © of EyeRounds.org of The University of Iowa. Permissions obtained from executive director and editor of Eyerounds.org.
- ↑ Hamel, Christian. Retinitis Pigmentosa. Orphanet Journal of Rare Diseases 2006 1:40 doi:10.1186/1750-1172-1-40.This is an Open Access article distributed under the terms of the Creative Commons Attribution License, which permits unrestricted use, distribution, and reproduction in any medium, provided the original work is properly cited.© 2006 Hamel; licensee BioMed Central Ltd.
- ↑ Gerardo Garcia-Aguirre, MD. Myopic Degeneration. Retina Image Bank. Oct 3, 2013; Image Number 10884. © The American Society of Retina Specialists. Permission for use obtained from Director of Retina Image Bank and Members Section of the ASRS. This image was originally published in the Retina Image Bank.
- ↑ Homayoun Tabandeh, MD. Ocular Albinism. Retina Image Bank. March 3, 2014; Image Number 14632. © The American Society of Retina Specialists. Permission for use obtained from Director of Retina Image Bank and Members Section of the ASRS. This image was originally published in the Retina Image Bank.
- ↑ MacDonald IM, Mah DY, Ho YK, Lewis RA, Seabra MC. A practical diagnostic test for choroideremia. Ophthalmology. 1998;105(9):1637-1640.
- ↑ Lee TK, McTaggart KE, Sieving PA, et al. Clinical diagnoses that overlap with choroideremia. Canadian journal of ophthalmology. Journal canadien d'ophtalmologie. 2003;38(5):364-372; quiz 372.
- ↑ Zhu, Li, et al. "Diagnosis for choroideremia in a large Chinese pedigree by next‑generation sequencing (NGS) and non‑invasive prenatal testing (NIPT)." Molecular medicine reports 15.3 (2017): 1157-1164.
- ↑ Bainbridge JW, Smith AJ, Barker SS, et al. Effect of gene therapy on visual function in Leber's congenital amaurosis. The New England journal of medicine. 2008;358(21):2231-2239.
- ↑ Maguire AM, Simonelli F, Pierce EA, et al. Safety and efficacy of gene transfer for Leber's congenital amaurosis. The New England journal of medicine. 2008;358(21):2240-2248.
- ↑ Hauswirth WW, Aleman TS, Kaushal S, et al. Treatment of leber congenital amaurosis due to RPE65 mutations by ocular subretinal injection of adeno-associated virus gene vector: short-term results of a phase I trial. Human gene therapy. 2008;19(10):979-990.
- ↑ Lukashev AN,Zamyatnin AA, Jr. Viral Vectors for Gene Therapy: Current State and Clinical Perspectives. Biochemistry Biokhimiia. 2016;81(7):700-708.
- ↑ 78.0 78.1 78.2 78.3 Tolmachova T, Tolmachov OE, Barnard AR, et al. Functional expression of Rab escort protein 1 following AAV2-mediated gene delivery in the retina of choroideremia mice and human cells ex vivo. Journal of molecular medicine (Berlin, Germany). 2013;91(7):825-837.
- ↑ Zinkernagel MS, MacLaren RE. Recent advances and future prospects in choroideremia. Clinical ophthalmology (Auckland, N.Z.). 2015;9:2195-2200.
- ↑ Bennett J, Maguire AM, Cideciyan AV, et al. Stable transgene expression in rod photoreceptors after recombinant adeno-associated virus-mediated gene transfer to monkey retina. Proceedings of the National Academy of Sciences of the United States of America. 1999;96(17):9920-9925.
- ↑ 81.0 81.1 81.2 Edwards TL, Jolly JK, Groppe M, et al. Visual Acuity after Retinal Gene Therapy for Choroideremia. The New England journal of medicine. 2016;374(20):1996-1998.
- ↑ Xue, Kanmin, et al. "Beneficial effects on vision in patients undergoing retinal gene therapy for choroideremia." Nature medicine 24.10 (2018): 1507-1512.
- ↑ MacLaren, Robert E., et al. "Retinal gene therapy in patients with choroideremia: initial findings from a phase 1/2 clinical trial." The Lancet 383.9923 (2014): 1129-1137.
- ↑ Davis, Janet L. "The blunt end: surgical challenges of gene therapy for inherited retinal diseases." American journal of ophthalmology 196 (2018): xxv-xxix.
- ↑ MacLaren, Robert E., et al. "Retinal gene therapy in patients with choroideremia: initial findings from a phase 1/2 clinical trial." The Lancet 383.9923 (2014): 1129-1137.
- ↑ Schaffrath, Kim, et al. "One-year safety and performance assessment of the Argus II retinal prosthesis: a postapproval study." JAMA ophthalmology 137.8 (2019): 896-902.
- ↑ Moosajee, Mariya, et al. "Functional rescue of REP1 following treatment with PTC124 and novel derivative PTC-414 in human choroideremia fibroblasts and the nonsense-mediated zebrafish model." Human molecular genetics 25.16 (2016): 3416-3431.
- ↑ Lima VC, Rosen RB, Farah M. Macular pigment in retinal health and disease. International journal of retina and vitreous. 2016;2:19.
- ↑ 89.0 89.1 89.2 Duncan JL, Aleman TS, Gardner LM, et al. Macular pigment and lutein supplementation in choroideremia. Experimental eye research. 2002;74(3):371-381.
- ↑ Genead MA, McAnany JJ, Fishman GA. Topical dorzolamide for treatment of cystoid macular edema in patients with choroideremia. Retina (Philadelphia, Pa.). 2012;32(4):826-833.
- ↑ Huckfeldt RM,Comander J. Management of Cystoid Macular Edema in Retinitis Pigmentosa. Seminars in ophthalmology. 2016:1-9.
- ↑ Liew G, Moore AT, Webster AR, Michaelides M. Efficacy and prognostic factors of response to carbonic anhydrase inhibitors in management of cystoid macular edema in retinitis pigmentosa. Investigative ophthalmology & visual science. 2015;56(3):1531-1536.
- ↑ Yuzbasioglu E, Artunay O, Rasier R, Sengul A, Bahcecioglu H.Intravitreal bevacizumab (Avastin) injection in retinitis pigmentosa. Current eye research. 2009;34(3):231-237.
- ↑ Melo GB, Farah ME, Aggio FB. Intravitreal injection of bevacizumab for cystoid macular edema in retinitis pigmentosa. Actaophthalmologica Scandinavica. 2007;85(4):461-463.
- ↑ 95.0 95.1 Edwards TL, Groppe M, MacLaren RE. Outcomes following cataract surgery in choroideremia. Eye (London, England). 2015;29(4):460-464.