Intracranial Pressure and Glaucoma
All content on Eyewiki is protected by copyright law and the Terms of Service. This content may not be reproduced, copied, or put into any artificial intelligence program, including large language and generative AI models, without permission from the Academy.
Intracranial Pressure and Glaucoma
Introduction
Glaucoma describes a group of conditions in which there is characteristic cupping of the optic disc with corresponding visual field defects due to retinal ganglion cell loss. It is the leading cause of irreversible blindness worldwide. Non-modifiable risk factors for glaucoma include age, ethnicity, corneal thickness, and genetic history.[1] The most recognized modifiable risk factor is intraocular pressure (IOP), and as such, most available glaucoma therapies focus on the reduction of IOP to reduce progression of glaucomatous damage and visual field loss.[2]However, further understanding of etiologies and mechanisms causing glaucomatous damage need to be explored as not all people with ocular hypertension develop glaucoma and glaucoma can develop in cases of normal IOP (<22 mm Hg), classified under normal tension glaucoma (NTG).[3]
Lamina Cribrosa
The lamina cribrosa (LC) is a mesh-like structure in the posterior portion of the sclera that allows optic nerve fibers to leave the eye. Anterior to the LC is the interior of the eye, and posterior to the LC is the optic nerve surrounded by the meninges, all within the optic sheath. The cerebrospinal fluid within the subarachnoid space surrounding the optic nerve is continuous with the subarachnoid space surrounding the brain and spinal cord.[4][5] Acting on the LC are the opposing IOP and intracranial pressure (ICP). Recently, many studies have demonstrated that ICP can have an important role in structural damage to the optic nerve. Several studies reported significantly lower ICP in subjects with glaucoma compared with healthy subjects. [6][7]Furthermore, significantly higher ICP was recorded in subjects with ocular hypertension (no functional glaucomatous damage despite elevated IOP) compared with healthy eyes. [6][8] In an animal model, extended reduction in ICP resulted in ocular neural tissue loss in half of the monkeys. [9] Zhang and colleagues demonstrated similar changes in axonal transport and morphology and immuno-histochemical staining in rats who had elevated IOP and decreased ICP. [6] These findings suggest that both IOP and ICP may play an important role in the disease process.
Translaminar Pressure Gradient
The translaminar pressure gradient (TLPG) created at the LC level between ICP and IOP could be the major determinant for the damage to the optic nerve at the LC level.[10] The TLPG is estimated to be 20 to 33 mm Hg/mm on average in humans. [7][8] Jonas and colleagues stated that the main factors that contribute to the gradient are LC thickness, IOP, and the pressure of retrolaminar tissue.[10][11] The TLPG may be defined as the difference between IOP and ICP per unit thickness of the LC (TLPG = (IOP/ICP) /thickness of LC).[12] The LC is considered to be the primary site of axonal damage in glaucoma, associated with the distortion of axoplasmic flow in the axons of ganglion cells. A posterior movement of LC and increased depth are described for the patients with glaucoma. It has been shown that the increased depth of the anterior surface of the LC (ASLC) precedes the structural damage and the loss of the nerve fiber layer. In the future, ASLC depth and the translaminar gradient may be considered as additional and earlier information for the diagnosis of glaucoma.[13][14][15][16] The swept source (SS) optical coherence tomography (OCT) and enhanced depth imaging (EDI) have permitted these evaluations.[17]
CSF Theory of Glaucoma
Fleishman and Berdahl have postulated the CSF theory of glaucoma, which defines that the balance between the two pressures (ICP and IOP) determines the TLPG. If the ICP is reduced or the IOP is increased, the TLPG will increase, and the damage to the LC will appear, with an increase in ASLC depth and cupping of the optic disk. The reverse effect of the CSF theory can be seen in clinical conditions like idiopathic intracranial hypertension (IIH) and ocular hypotony. These conditions cause an unbalanced anteriorly directed force either due to an elevated ICP and normal IOP (IIH) or a normal ICP and low IOP (ocular hypotony). The net result is a negative cupping effect or swollen optic disc. Patients with IIH may tend to have ocular hypertension to counterbalance the increased ICP, maintaining a homeostatic gradient. It has been proposed that a clinically increased IOP may be a treatment option for patients with IIH.[18] (Fig.1) Health of the optic nerve head is maintained by a homeostatic pressure difference between the posteriorly directed IOP and an anteriorly directed ICP.
Another hypothesis explaining the correlation of low ICP with glaucoma progression is that CSF flow to the optic nerve is reduced or cut off when ICP is low, or IOP is high. Several studies in animal and human subjects did demonstrate a reduction of CSF flow to the optic nerve in glaucoma and NTG subjects. [19][20][21]
Evidence Supporting Glaucoma Developing from Reduced Intracranial Pressure
Several studies have demonstrated a correlation between low ICP and glaucoma. Berdhan and colleagues assessed the ICP in individuals with and without primary-open angle glaucoma (POAG). They reported that the mean ICP was significantly lower in POAG patients (11.2 mmHg) compared to controls (11.8 mmHg) (p<0.0001).[22] Furthermore, the mean ICP was significantly lower in NTG patients (8.7 mmHg) compared to POAG patients (9.1 mmHg).[23]
Two years later, Ren and colleagues conducted a prospective study evaluating ICP in POAG, NTG patients, and normal controls. They showed that the mean ICP was significantly lower in NTG (9.5 mmHg) compared to POAG patients (11.7 mmHg) and normal controls (12.9 mmHg).[24]
Siaudvytyte and colleagues investigated correlations between TLPG and the presence of structural and functional manifestations of glaucoma. They demonstrated the POAG had a significantly higher TLPG. Additionally, they showed that in the NTG, higher TLPG was associated with decreased neuroretinal rim area.[25]
Morgan et al. concluded that CSF pressure was the major determinant of the post-laminar pressure, with the effect of altering the CSF pressure being biomechanically equivalent to altering the IOP. These factors could potentially influence the development of NTG or the susceptibility of the optic nerve to ocular hypertension.[26]
Yablonski et al. experimentally demonstrated that glaucoma-like cupping and axonal swelling in cat eyes could be induced by decreasing ICP and additionally counteracted the changes by concurrently decreasing IOP. It is noteworthy that the ICP decreases with age, and this may partly explain the higher prevalence of glaucoma with old age.[27]
However, it is essential to acknowledge that, despite the growing body of evidence supporting the association between low ICP and glaucoma development, there are some studies with conflicting results. For instance, a study by Linden and colleagues prospectively investigated the ICP in NTG patients versus normal controls, and they reported no significant difference between the two groups.[28]
Normal Pressure Hydrocephalus and Glaucoma
Chang and Singh conducted a retrospective evaluation of the prevalence of glaucoma in patients with normal pressure hydrocephalus (NPH), whether they had an intracranial shunt or not. Their findings revealed a significant three-fold higher prevalence of glaucoma in NPH patients (18.1%) compared to age-matched non-NPH controls (5.6%) (p=0.02).
The authors hypothesized that NPH patients might exhibit an increased neural susceptibility to pressure-related damage. This increased susceptibility could potentially explain the development of neurological symptoms despite normal ICP, alongside the onset of glaucoma.[29] Another theory is that some of these patients received ventriculo-peritoneal shunts (VPS), which effectively lowered their ICP, resulting in increased TLPG and subsequent glaucomatous damage.[30] This theory gains support from a study by Gallina and colleagues, who evaluated NPH who had undergone VPS six months or more prior. They reported that nine out of 22 NPH patients developed new-onset NTG after shunt placement.
Furthermore, they demonstrated that the duration of exposure to low ICP was a significant risk factor for glaucoma development.[31] A subsequent follow-up study by the same group showed that two additional patients in the initial non-NTG glaucoma group developed NTG, one at 9.5 years and another 4 years from the time of shunt placement. This finding increased the number of NTG patients after VPS to 11 (50% of the initial cohort).[32]
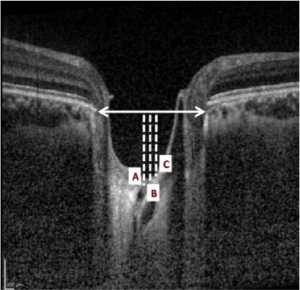
ASLC depth and Intracranial Hypotension
Soares et al. analyzed the LC depth and the optic nerve in patients with Intracranial Liquor Hypotension Syndrome (ILHS).[33] They found that the ASLC depth was significantly higher in the group of patients with ILHS compared to the control group. (Fig.2) The depth of ASLC was defined as the distance between the plan of Bruch’s membrane opening (BMO) and ASLC (Fig.3).
This study demonstrates that the TLPG is a determinant in the structure of the LC. Low ICP associated with a high TLPG leads to an increased LC depth similar to that observed in glaucoma patients. This was the first study about the evaluation of LC and optic nerve of patients with ILHS that emphasized the importance of ICP and TLPG in the structure of LC and optic nerve.
Challenges
The evaluation of TLPG and ICP will be a future tool used to complement the study of the optic nerve and to evaluate patients with glaucoma. However, there are many factors that have not been well explained: 1) it is not clear if ICP evaluated by lumbar puncture considers the presence of orbital septum that can limit the flux of fluid in the orbit; 2) the fluid dynamics have not been well defined and the role of postural changes and patient activity in the evaluation of ICP is not clear; 3) the better method to evaluate ICP (invasive or noninvasive) has not been defined.[34]
Currently, ICP is often measured via lumbar puncture. Although lumbar puncture is invasive, it has been shown to accurately represent ICP.[35] Efforts to measure ICP noninvasively have been explored and may be considered when lumbar puncture is contraindicated, but have not proven to be as reliable and accurate as lumbar puncture.[36][37] When combined with the variability of measurements in regards to postural and diurnal changes, the fluctuation in measurements may challenge the reliability of studies assessing for differences in ICP and TLPG, as the pressures between glaucomatous and non glaucomatous patients can be quite close at a few mm Hg apart.
Nevertheless, the relationship between ICP and IOP is not well understood, and some studies have also shown a lack of correlation between ICP and IOP.[38]
Clinical Implications
Considering all retrospective, prospective, and experimental data, we have to consider the importance of ICP in diagnosing and treating glaucoma. Also, the ophthalmologist can now look for signs of glaucoma in patients with subtle neurological symptoms of low ICP, like postural headaches. The physiologic balance between these two pressures is important to the health of retinal ganglion cells and their axons. Dysregulation of this process may have an important role in the pathogenesis of glaucoma.
In conclusion, it is essential to consider the TLPG and ICP in the evaluation of patients with glaucoma.
References
- ↑ Quigley H. Glaucoma. Lancet 2011;377:1367-77.
- ↑ Vidal-Sanz M, Salinas-Navarro M, Nadal-Nicolas F, et al. Understanding glaucomatous damage: Anatomical and functional data from ocular hypertensive rodent retinas. Progress in Reginal and Eye Research 2012;31:1-27.
- ↑ MA K, DK H, EJ H, et al. The Ocular Hypertension Treatment Study: a randomized trial determines that topical ocular hypotensive medication delays or prevents the onset of primary open-angle glaucoma. Arch Ophthalmol 2002;120(6):701-13.
- ↑ WH M, DY Y, RL C, et al. The influence of cerebrospinal fluid pressure on the lamina cribrosa tissue pressure gradient. Invest Ophthalmol Vis Sci 1995;36(6):1163-72.
- ↑ WH M, DY Y, VA A, et al. The correlation between cerebrospinal fluid pressure and retrolaminar tissue pressure. Invest Ophthalmol Vis Sci 1998;39(8):1419-28.
- ↑ 6.0 6.1 6.2 Zhang Z, Liu D, Jonas JB, et al. Axonal transport in the rat optic nerve following short-term reduction in cerebrospinal fluid pressure or elevation in intraocular pressure. Invest Ophthalmol Vis Sci. 2015;56:4257–4266.
- ↑ 7.0 7.1 Morgan WH, Yu DY, Alder VA, et al. The correlation between cerebrospinal fluid pressure and retrolaminar tissue pressure. Invest Ophthalmol Vis Sci. 1998;39:1419–1428.
- ↑ 8.0 8.1 Balaratnasingam C, Morgan WH, Johnstone V, et al. Histomorphometric measurements in human and dog optic nerve and an estimation of optic nerve pressure gradients in human. Exp Eye Res. 2009;89:618–628.
- ↑ Yang D, Fu J, Hou R, et al. Optic Neuropathy Induced by Experimentally Reduced Cerebrospinal Fluid Pressure in Monkeys. Invest Ophthalmol Vis Sci. 2014. January 13;55(5):3067–73.
- ↑ 10.0 10.1 Downs JC and Girkin CA. Lamina cribrosa in glaucoma. Curr Opin Ophthalmol 2017, 28:113–119
- ↑ Jonas JB, Berenshtein E, Holbach L. Lamina cribrosa thickness and spatial relationships between intraocular space and cerebrospinal fluid space in highly myopic eyes. Invest Ophthalmol Vis Sci. 2004;45:2660–2665.
- ↑ Berdahla J, Allingham R. Intracranial pressure and glaucoma. Current Opinion in Ophthalmology 2010;21:106-11.
- ↑ Seo JH, Kim T-W, Weinreb RN. Lamina cribrosa depth in healthy eyes. Invest Ophthalmol Vis Sci. 2014;55:1241–1250. DOI:10.1167/iovs.13-12536
- ↑ Kim YW, Jeoung JW, Kim DW, et al. (2016) Clinical assessment of lamina cribrosa curvature in eyes with primary open-angle glaucoma. PLoS ONE 11(3): e0150260. doi:10.1371/journal.pone.0150260
- ↑ Kim DW, Jeoung JW, Kim YW, et al. Prelamina and lamina cribrosa in glaucoma patients with unilateral visual field loss. Invest Ophthalmol Vis Sci. 2016;57:1662–1670. DOI:10.1167/iovs.15-18453
- ↑ Feola AJ, Myers JG, Raykin J, et al. Finite element modeling of factors influencing optic nerve head deformation due to intracranial pressure. Invest Ophthalmol Vis Sci. 2016;57:1901–1911. DOI:10.1167/iovs.15-17573
- ↑ Sigal IA, Wang B, Strouthidis NG, et al. Recent advances in OCT imaging of the lamina cribrosa. Br J Ophthalmol. 2014;98(Suppl 2):ii34–ii39. doi:10.1136/bjophthalmol-2013-304751
- ↑ Chowdhury UR, Fautsch M. Intracranial Pressure and its relationship to glaucoma: Current understanding and future directions. Med Hypothesis Discov Innov Ophthalmol. 2015; 4(3): 71-80.
- ↑ Mathieu E, Gupta N, Paczka-giorgi LA, et al. Reduced cerebrospinal fluid inflow to the optic nerve in glaucoma. Invest Ophthalmol Vis Sci. 2018;59:5876–5884.
- ↑ Boye , Montali M, Miller NR, et al. Flow dynamics of cerebrospinal fluid between he intracranial cavity and the subarachnoid space of the optic nerve measured ith a diffusion magnetic resonance imaging sequence in patients with normal tension glaucoma. Clin Experiment Ophthalmol. 2018;46:511–518.
- ↑ Jaggi P, Harlev M, Ziegler U, et al. Cerebrospinal fluid segregation optic neuropathy: an experimental model and a hypothesis. Br J Ophthalmol. 010;94:1088–1093.
- ↑ Berdahl JP, Allingham RR, Johnson DH. Cerebrospinal fluid pressure is decreased in primary open-angle glaucoma. Ophthalmology. 2008;115:763–768.
- ↑ Berdahl JP, Fautsch MP, Stinnett SS, Allingham RR. Intracranial pressure in primary open angle glaucoma, normal tension glaucoma, and ocular hypertension: a case-control study. Invest Ophthalmol Vis Sci. 2008;49:5412–5418.
- ↑ Ren R, Jonas JB, Tian G, et al. Cerebrospinal fluid pressure in glaucoma: a prospective study. Ophthalmology. 2010;117:259–266.
- ↑ Siaudvytyte L, Januleviciene I, Ragauskas A, Bartusis L, Siesky B, Harris A. Update in intracranial pressure evaluation methods and translaminar pressure gradient role in glaucoma. Acta Ophthalmol. 2015;93:9–15.
- ↑ Morgan-Davies J, Taylor N, Hill AR, et al. Three dimensional analysis of the lamina cribrosa. Br J Ophthalmol. 2004; 88(10): 1299–1304.
- ↑ Fleischman D, Berdahl J, Zaydlarova J et al. Cerebrospinal Fluid Pressure Decreases with Older Age. PLoS One. 2012; 7(12): e52664
- ↑ Lindén C, Qvarlander S, Jóhannesson G, et al. Normal-tension glaucoma has normal intracranial pressure: a prospective study of intracranial pressure and intraocular pressure in different body positions. Ophthalmology. 2018;125:361–368.
- ↑ Chang TC, Singh K. Glaucomatous disease in patients with normal pressure hydrocephalus. J Glaucoma. 2009;18:243– 246.
- ↑ Lee MS, McCulley TJ, Lee AG, Van Stavern GP. Does Intracranial Pressure Influence the Development of Glaucoma? J Neuroophthalmol. 2023 Sep 1;43(3):423-429.
- ↑ Gallina P, Savastano A, Becattini E, Orlandini S, Scollato A, Rizzo S, Carreras G, Di Lorenzo N, Porfirio B. Glaucoma in patients with shunt-treated normal pressure hydrocephalus. J Neurosurg. 2018 Oct;129(4):1078-1084.
- ↑ Gallina P, Savastano A, Buzzi M, Angelini L, Miele A, Rizzo S, Scollato A, Caini S, Porfirio B. Normal tension glaucoma in CSF-shunted normal pressure hydrocephalus patients. An extended follow-up. Eye (Lond). 2023 Jan;37(1):183-184.
- ↑ Soares A, Lopes N, Morgado G. Study of lamina cribrosa depth and optic nerve in patients with spontaneous intracranial hypotension. Eur. J Ophthalmol. 2019 Nov; 29(6).
- ↑ Furlanetto RL, Park SC, Damle UJ, et al. Posterior displacement of the lamina cribrosa in glaucoma: in vivo interindividual and intereye comparisons. Invest Ophthalmol Vis Sci. 2013;54: 4836–4842
- ↑ N L, LO K, AT B, et al. CSF pressure assessed by lumbar puncture agrees with intracranial pressure. Neurology 2007;68(2):155-8.
- ↑ Bruce B. Noninvasive assessment of cerebrospinal fluid pressure. J Neuroophthalmol 2014;34(3):288-94.
- ↑ X Z, JE M, BJ I, et al. Invasive and noninvasive means of measuring intracranial pressure: a review. Physiol Meas 2017;38(8):R143-R82.
- ↑ Pircher A, Remonda L, Weinreb RN, et al. Translaminar pressure in Caucasian normal tension glaucoma patients. Acta Ophthalmol. 2017;95(7):e524-e531.