Spaceflight-Associated Neuro-Ocular Syndrome (SANS)
All content on Eyewiki is protected by copyright law and the Terms of Service. This content may not be reproduced, copied, or put into any artificial intelligence program, including large language and generative AI models, without permission from the Academy.
Disease Entity
Disease
Spaceflight associated Neuro-Ocular Syndrome (SANS)—formerly called visual impairment and intracranial pressure (VIIP) syndrome[1]—is a constellation of findings and symptoms that have been found in astronauts who have undergone long duration space flight (LDSF) missions in microgravity environments (e.g., International Space Station (ISS)).[2] SANS enterprises a constellation of signs that are only partially and problematically elucidated solely by increased intracranial pressure (ICP). Therefore, SANS represents a nomenclatural modification to reflect the relative uncertainty of the exact etiology & pathogenesis.[3] [4]
Etiology
Known to be a multifaceted mechanism consisting of components of the theory of cephalad fluid shift, compartmentalization & alternative compartmentalization theories, increased intracranial pressure, differential translaminar pressure gradients, aberrancies in lymphatic drainage systems, intracerebral volume shifts, orbital and cerebral arterial and vortex vein drainage alterations, cyanocobalamin- & folate-dependent one-carbon pathway variances, choroidal volume expansion, and ambient hypercapnic environments onboard the ISS may contribute to the SANS constellation.[5] Due to limitations in sample sizes, the inherent complexity in characterizing SANS-defining signs, and analysis outcomes demonstrating lack of sufficient evidence to pinpoint a single well-evidenced etiology for SANS, it is likely that SANS represents a multi-factorial origin with key clinical highlights from multiple hypothesized theories.[6] Only roughly 12 astronauts enter spaceflight annually, at a rate of about 3 astronauts per 3 months; this places a limitation in sample size when attempting to characterize SANS. [5]
Risk Factors
The primary risk factor for SANS is extended exposure to the unique microgravity environment of LDSF (e.g. ISS).[2] Subjective symptoms from SANS follow a dose dependent response with up to 23% experiencing changes in near distance visual acuity after short duration shuttle missions and up to 48% reporting near visual acuity changes after LDSF ISS missions.[2] In addition, up to 45% of astronauts flying missions greater than 30 days demonstrate ocular abnormalities through fundus examination and orbital and brain imaging[7] with or without the presence of subjective symptoms. Associated risk factors predisposing to increased ICP during LDSF, and consequently SANS, include high salt diets, intensive resistive exercise, liability to inflated ambient CO2 concentrations, lack of good nutrition[8], and biochemical aberrancies in cyanocobalamin- & folate-dependent 1-carbon transfer pathways.[5] Interestingly, astronauts with ophthalmic-related symptoms were shown to possess decreased folate serum levels during spaceflight.[8] No discrepancies were observed in vitamin B12 serum concentrations during spaceflight; however, serum concentrations of methylmalonic acid were noticeably greater post-LDSF in astronauts with reported ophthalmic-related symptoms.[8]
Pathophysiology
Critical developments in our understanding of SANS have laid the foundation for several competing mechanisms. While all proposed mechanisms attempt to reconcile the classical constellation of SANS-defining signs, including hyperopic shift, choroidal & retinal folds, cotton-wool spots, optic disc edema, optic nerve sheath distension, and globe flattening)[3], only few can harmonize a sound solution without intruding on closely-related disease processes.[5] Although the exact etiology of SANS is still unknown it is believed that cephalad fluid shifts to the head, neck and orbits occur in microgravity environments due to impaired gravity assisted lymphatic, CSF and vascular drainage. These cephalad fluid shifts may cause increased hydrostatic pressure in the brain (intracranial pressure)[2] and orbit (optic nerve sheath). Two primary hypotheses have emerged. First, cephalad fluid shifts may lead to increased intracranial volume and/or pressure. This elevated CSF pressure around the brain may be transferred down the ON sheaths to the orbit and cause disc edema as well as globe flattening. Some studies have demonstrated that cerebral autoregulation is preserved during spaceflight[9] yet venous stasis in both the jugular and femoral veins has been observed during spaceflight and suggests venous congestion[10] which might impact CSF outflow and can create a terrestrial IIH like picture.[10] This congestion may also inhibit vortex vein drainage and cause choroidal thickening that could add to axial shortening and the observed hyperopic shift.[2] . The absence of classical symptoms as seen in terrestrial IIH, including headache, tinnitus, transient visual obscurations, nausea, vomiting and the lack of data on inflight CSF opening pressure make this “IIH like” theory arguable.
A second hypothesis is that SANS is due to microgravity induced changes in the physiology of CSF and subtle variations in interpersonal physiology of flow and drainage within the optic nerve sheath itself. It has been proposed that the optic nerve sheath may possibly create a one-way valve-like system, causing the CSF to become compartmentalized within the optic nerve sheath without elevating the CSF around the brain.[5] CSF infusion studies show linear optic nerve sheath distention up to a saturation point which varies from person to person[1][11]and might explain the asymmetric findings in both IIH[12] and SANS. CSF flow within the obit is generally understood as related to inflow and outflow of CSF but postural/gravitational effects, ventricular pulsations and pulse pressure of the choroid plexus may also have a role.[12]. CSF dynamics were tested by modeling of spaceflight environments via head down simulation and MG studies showcase non-appreciable changes in cerebral arterial diameter & blood flow velocities; however, autoregulatory behavior of middle cerebral artery diameters is not clearly understood.[5]
In addition, elevated pressures in the cerebral venous vasculature could lead to relatively reduced functionality of the vortex vein drainage system, the principle choroidal drainage site. Despite this reduced functionality, some studies have demonstrated that cerebral autoregulation is preserved during spaceflight[9] yet venous stasis in both the jugular and femoral veins has been observed during spaceflight and suggests venous congestion[10] In addition to drainage into venous vasculature, lymphatic drainage is believed to play a role as seen by the presence of India ink in lymphatics after injections into the optic nerve subarachnoid space.[13]Contrast infusion studies in IIH patients[12] by Killer et al demonstrate compartmentalization of the optic nerve sheath which is further supported by the presence of high to low chemical gradients between the optic nerve and intracranial subarachnoid spaces.[14] The combination of venous and lymphatic stasis may contribute to an optic nerve sheath compartment syndrome in the presence of only borderline increases to ICP. A possible third explanation, by some researchers, include the choroidal expansion secondary to cephalad shifting of cerebral fluids as a plausible adjunctive mechanism explaining retinal & choroidal folds, loss of convexity of the posterior globe, and subsequent hyperopic refractive shifting.[5] This congestion may also inhibit vortex vein drainage and cause choroidal thickening that could add to axial shortening and the observed hyperopic shift.[2]
Although spaceflight-associated radiation exposure is mitigated to a significant extent by Earth’s magnetosphere, exploration missions beyond low Earth orbit, where the ISS is currently located, may predispose astronauts to clinically detrimental amounts of radiation, as seen in the lens, retina and aortic vasculature in radiation-exposed murine models.[15] Radiation exposures of 20 Gy showcased newly-evolved inflammation of the cerebral parenchyma and dose-dependent impairment of the brain-blood barrier (BBB).[3] Modeling of this process demonstrated reasonable suspicion of ensuing development of elevated ICP.[3].Post-terrestrial radiation exposure is documented in association with cotton-wool spots (CWS) development, which provides some level of insight into the possible role space-flight associated radiation exposure may play in the development of CWS and relevant SANS signs.[5] Controversial discussions regarding the influence of hypercapnic environments in the development of increased ICP is unyielding.[5] With astronauts onboard the ISS exposed to carbon dioxide (CO2) concentrations approximately 10 times greater than Earth normal, importance towards the exact delineation of elevated CO2 levels play in the development of SANS should not be underestimated.[5]
Diagnosis
Physical examination
-Visual acuity= Increased hyperopic sphere.[2]
-Cycloplegic and manifest refraction= normal to near normal vision.
-Fundoscopic exam= Disc edema, cotton wool spots, glutting of the superior and inferior nerve fibers[3], and choroidal folds.[2][7]. Asymptomatic cases of bilateral, asymmetrical optic disc edema can occur in SANS.[3]
-Optical coherence tomography-CNN
(Tonometry assessments for IOP are not reliable indicators for development of SANS, as dictated by NASA Lifetime Surveillance of Astronaut Health.[3])
Signs
Astronauts with SANS may have disc edema, choroidal folds, cotton wool spots, nerve fiber layer thickening on OCT, globe flattening and hyperopic shift.[2] While these signs may originate during spaceflight, no cases thus far have engendered a life-threatening or mission-endangering potential.<[3] When compared to IIH optic nerve edema, the optic nerve in SANS has linear areas of fold (vs concentric “Patons lines”) and the posterior fundus shows choroidal folds that may appear before retinal folds.[5] Additionally, reduction of optic nerve edema with directed alleviation of ICP etiologies in IIH is associated with residual optic nerve atrophy; this finding is not demonstrated in SANS.[5]
Symptoms
Symptoms include decreased near-visual acuity (hyperopic refractive shift), visual scotomas, headaches [2] and deterioration of distance.[3]The most frequent symptomatic complaint among astronauts experiencing SANS is decreased near vision caused by a hyperopic shift in vision of up to 1.5 diopters which can appear as early as after 3 weeks of microgravity exposure.[2] Self-reported SANS-related symptoms correlated with spaceflight time in a dose-dependent manner with 23% claiming disturbances in near-sightedness after short-duration space flight (SDSF) and up to 47% claiming similar symptoms after LDSF onboard the ISS.[2]
Despite bearing superficial similarities to terrestrial idiopathic intracranial hypertension, astronauts do not experience diplopia, pulsatile tinnitus or transient visual obscurations,[2] the common symptoms of IIH.[16] Less frequently observed symptoms also include visual scotomas[2] and headaches. No changes in best corrected visual acuity, color vision or other complaints have been noted to date.
Diagnostic procedures
Diagnostic procedures in astronauts experiencing SANS to date have included pre and post post MRI, pre-, intra-, and post flight OCT, orbital ultrasound, and occasional postflight lumbar punctures.[2] Findings from these imaging procedures may show structural correlates to the clinical findings of optic disc edema, globe flattening, dilation of the optic nerve sheath as well as thickening and tortuosity of the optic nerve.[2] MRI imaging may show increases to optic nerves sheath diameter and optic nerve diameter with “kinks” to the optic nerve visible as T2 hyper intensities (96%).[17][3]
Posterior globe flattening and pituitary dome concavity with posterior stalk deviation were also seen within the microgravity exposure cohorts.[17] Cephalad brain shift has also been demonstrated on post flight MRI. In flight diagnostic procedures are limited by technical limitations and equipment portability. Orbital ultrasound is used on the ISS and has detected qualitative globe flattening.[5]OCT and funduscopic examination are performed with remote data transmission to terrestrial Subject Matter Experts (e.g. Ophthalmologists)during missions and has demonstrated optic disc edema, chorioretinal folds, and cotton wool spots.[2][3] OCT Angiography (OCTA) has recently been introduced to the ISS and will provide more comprehensive, quantitative data on the changes in choroidal blood flow, which may increase the understanding of SANS and its cephalad fluid shift theory. [5]
Lumbar puncture and MRI have also been used to assess SANS but are limited to terrestrial use. Evaluation of SANS with MRI may show flattening of posterior globes, distended optic nerve sheaths, tortuous optic nerves and papilledema.[2] Lumbar punctures performed in the context of SANS typically demonstrate normal to borderline increased opening CSF pressures which have been documented as high as 28.5 cmH20 at 2 months after landing.[2] Lumbar puncture and MRI have also been used to assess SANS but are limited to terrestrial use. Evaluation of SANS with MRI may show flattening of posterior globes, distended optic nerve sheaths, tortuous optic nerves and papilledema.[2] Lumbar punctures performed in the context of SANS typically demonstrate normal to borderline increased opening CSF pressures which have been documented as high as 28.5 cmH20 at 2 months after landing.[2]. Remote ultrasound-guided lumbar puncture may prove beneficial and safe while in flight.[18] Many of these findings bear a similarity to terrestrial IIH; however, the findings in SANS frequently present asymmetrically[2] which is uncommon for IIH.[19]
Although lumbar punctures (LP) are readily performed terrestrially with minimal risk of procedure-associated adverse complications, discussions regarding the practicality of LP during spaceflight are limited by obvious operational challenges in spite of a perceived clinical utility in characterizing spaceflight ICP to a greater degree.[5] ICP condition surveillance utilizing population-based data is not currently possible as solely astronauts observing optic disk edema were retrieval of post-flight ICP measurements acquired from.[5] Additionally, LP in SANS may render normal opening pressure results, therefore its utility in diagnosing SANS is debated.[3] Clinical benefit of ultrasonographic measures available on the ISS have yet to be elucidated.[5].
Retinal and choroidal vessel microalterations as depicted in OCT- Angiography (OCTA) imaging demonstrate favorable insight into terrestrial retinal and choroidal disease processes.[5] While OCTA analysis of SANS development aboard the ISS awaits review, enhanced depth imaging (EDI) OCT, OCT2, and MultiColor Imaging (MCI) will likely provide an increase in discrepancy power for characterization of retinal, vitreous, and choroidal structures via enhanced digital resolution<[5] Heidelberg Spectralis “OCT2” is critically acclaimed for manufacturing more dense visualization of the optic nerve head, retina, and choroid with multi-color imaging (MCI) – a feature which will act as a competent substitute for routine spaceflight fundoscopic examination. Due to the non-invasive nature, industrial-grade resolution of posterior orbit structures, patient-consistency, and operator receptivity, the OCT2 imaging modality is the current diagnostic imaging test of choice for initial unmasking and observation of SANS.[5] [20]
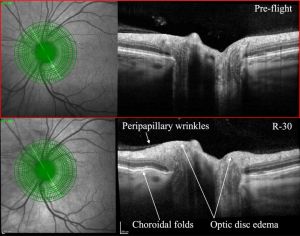
Contextual clarity in development of SANS-related posterior orbit microalterations may be discerned via Diffusion Tensor Imaging (DTI), an advanced MRI-based neuroimaging technique utilizing vector-dependent analysis of tissue water molecule movements to determine cerebral axonal organization. Masking of flight status to the interpreting neuroradiologist is important to reduce false-positive bias by conveniently overcompensating for clinically-insignificant posterior globe flattening in MRI imaging. While utilization of advanced imaging equipment will create provision for greater diagnostic clarity, future study designs should aim to incorporate masking.[5]
In addition, time-wise analysis of MRI neuroimaging of astronauts may provide utility in determining time-dependent progression and recovery in SANS.[5] Although the clinical picture of SANS is unique to the microgravity environment, similar OCT changes have been observed in head down tilt (HDT) bed rest studies on earth.[21] A 70-day trial of HDT bed rest found optic disc swelling and increased peripapillary retinal thickening, signs also seen in SANS. [21] These HDT bed rest studies, as well as HDT bed rest in the setting of hypercapnia, have become an increased interest in a potential terrestrial analog for studying SANS and its countermeasures.[5]
More recently, artificial intelligence aplication on a lightweight convolutional neural network (CNN) with an EfficientNet encoder that studies OCT images inflight and may detect SANS changes. Even if the OCT inflight datea is available, the increased in transmission latency as the spacecraft advances creates a delay of information send to Earth to be interpreted by an expert. One consideration is that there may be low computational capacity for this in the said spaceflight environment[22]
Laboratory test
Laboratory testing for enzymatic deficiencies in cyanocobalamin- and folate-dependent 1-carbon pathways may shed light on predisposition for SANS development in astronauts.[8]
Management
General treatment
SANS-directed therapeutics follow a multi-faceted countermeasure approach. These include aiding potential enzymatic defects in folate- & cyanocobalamin-dependent 1-carbon pathways, securing a relative decrease in TLPD via utility of swim goggles[5] and selective use of acetazolamide for reduction in ICP.[3] Additional treatment modalities includes ensuring proper nutritional supplementation during spaceflight<[8], targeted exercise regimes with selective preference for non-intensive resistive exercises[5], and simulation of earth-like gravitational environments during spaceflight.[5][3] Several pairs of “Space Anticipation Glasses” of varying powers are routinely deployed with ISS crewmembers in order to mitigate any hyperopic shifts experienced during the LDSF.[2] Considering the cephalad fluid shift seen in microgravity, some efforts have focused on simulating gravity by creating relative lower body negative pressure to mimic gravity.[23] Efforts have successfully decreased ICP but have yet to be applied during spaceflight.[23][24]
Medical therapy
Acetazolamide (Diamox Sequel) 500mg and 250mg for 6 and 2 weeks, respectively, may afford utility via decreases in cerebral pressures, as evidenced by post-LDSF lumbar puncture opening pressures in this case study.[3] Clinical support for administration of acetazolamide during spaceflight remains debatable.[3] TLPD assessments may provide the required clinical context for relief of optic disk edema via subsequent modifications in IOP and ICP.[3]
Among the non-medical therapy tried in the past[25] was an intake of traditional Chinese herbs as a countermeasure effect against weightlessness(-6° HDT used to simulate weightlessness). During bed rest, the near vision and IOP showed a wavelike decreases when herbs were taken. The effect of these herbs are not been tested in people with flight
Medical follow up
Follow up with visual field (VF) perimetry testing within 72-hours upon return from spaceflight can ascertain the contribution of optic disc edema, if present, to formation of blind spots.[3] In addition, assessment of OCT Retinal nerve fiber layer (RNFL) thickness at regular intervals post-spaceflight can aid in understanding the impact of chronic optic disc edema on RNFL pruning.[3] Signs of SANS may persist even after a return to normal earth gravity including the presence of mildly elevated CSF opening pressures[2] choroidal folds, and disc edema.[7][26].
Surgery
None
Complications
Certitude in permanence of SANS-associated visual acuity discrepancies remains uncertain; however, clear lack of consistency exists in time to reinstatement of normal visual function following presentation of SANS.[3][2] No permanent visual loss has yet occurred in any ISS crew members due to SANS.
Prevention
Because SANS results in structural and sometimes residual changes in vision, and because it increases as spaceflight duration increases, NASA has continued attention and investigation in this matter, however, it has been somewhat limited due to small sample size of humans on spaceflight. Therefore HDTBR studies on Earth continue to be important[27]
Prevention of SANS remains undetermined, however heightened efforts by NASA via an intensified occupational monitoring program for all active-duty astronauts, including specified attempts to characterize ICP-related signs: protrusion of the optic nerve head (ONH) ( shown to occur within the first 24 hours of increased ICP. Additionally, low salt diets, less intensive resistive exercise, and good nutrition may delay the development of SANS as explained above. [3] There have been some attempts to lower ICP without affecting cerebral perfusion such as negative pressure suits(specially inferior half of body up to 20mmHg) and thigh cuffs[28]. To increase IOP, there has been trials of artificial gravity and googles (that minimally increased IOP and translaminar pressure). Additionally, although the effect of CO2 has not yet been statistically proven, the NASA has reduced International Space Station ambient CO2. [29]
In recent studies[30] , by performing lower body negative pressure (LBNP) overnight, there hydrostatic gradients were reintroduced, therefore they were hemodynamically stable and choroid engorgement was attenuated. Therefore, at the first ocular remodeling finding, LBNP may be indicated.
Prognosis
Although some ocular structural changes from SANS may persist for years after spaceflight, including globe flattening, choroidal folds, and hyperopic shifts, no crew member has yet experienced permanent vision loss after the spaceflight.[5][3]
Questionnaire analysis of 300 astronauts demonstrated alterations in normal acuity failing exclusivity in astronauts with prior LDSF, with 29% of astronauts participating in SDSF reporting negative adjustments in near-visual acuity. Case reports illustrate persistence of post-spaceflight choroidal folds for at least 3 years after spaceflight.[3] Optic disc swelling has persisted in some up to 2 years after mission.[26]. Permanent increases in laxity of the collagenous structures of the eye and orbit including the trabecular fibers of the optic nerve sheath during LDSF may contribute to persistent signs of optic nerve sheath distension and retinal and choroidal folds upon return from spaceflight.[5]
Additional Resources
References
- ↑ 1.0 1.1 Wostyn P, Deyn PP De. Optic Nerve Sheath Distention as a Protective Mechanism Against the Visual Impairment and Intracranial Pressure Syndrome in Astronauts. 2017;(September 2017):2017-2020. doi:10.1167/iovs.17-22600
- ↑ 2.00 2.01 2.02 2.03 2.04 2.05 2.06 2.07 2.08 2.09 2.10 2.11 2.12 2.13 2.14 2.15 2.16 2.17 2.18 2.19 2.20 2.21 2.22 2.23 2.24 Mader TH, Gibson CR, Pass AF, et al. Optic Disc Edema, Globe Flattening, Choroidal Folds, and Hyperopic Shifts Observed in Astronauts after Long-duration Space Flight. Ophthalmology. 2011;118(10):2058-2069. doi:10.1016/j.ophtha.2011.06.021
- ↑ 3.00 3.01 3.02 3.03 3.04 3.05 3.06 3.07 3.08 3.09 3.10 3.11 3.12 3.13 3.14 3.15 3.16 3.17 3.18 3.19 3.20 3.21 3.22 Stenger M.B., Tarver W.J., Brunstetter T.et al. Risk of Spaceflight Associated Neuro-ocular Syndrome (SANS). NASA Human Research Program Human Health Countermeasures Element https://humanresearchroadmap.nasa.gov/evidence/reports/SANS.pdf NASA Johnson Space Center. 2017; (Available at:) https://humanresearchroadmap.nasa.gov/evidence/reports/SANS.pdf?rnd=0.434276635495143
- ↑ Yang JW, Song QY, Zhang MX, et al. Spaceflight-associated neuro-ocular syndrome: a review of potential pathogenesis and intervention. Int J Ophthalmol. 2022;15(2):336-341. Published 2022 Feb 18. doi:10.18240/ijo.2022.02.21
- ↑ 5.00 5.01 5.02 5.03 5.04 5.05 5.06 5.07 5.08 5.09 5.10 5.11 5.12 5.13 5.14 5.15 5.16 5.17 5.18 5.19 5.20 5.21 5.22 5.23 5.24 5.25 5.26 5.27 Lee, A.G., Mader, T.H., Gibson, C.R. et al. Spaceflight associated neuro-ocular syndrome (SANS) and the neuro-ophthalmologic effects of microgravity: a review and an update. npj Microgravity 6, 7 (2020). https://doi.org/10.1038/s41526-020-0097-9
- ↑ National Aeronautics and Space Administration – Human Research Program – Human Health Countermeasures Element. Risk of Spaceflight Associated Neuro-ocular Syndrome (SANS). Humanresearchroadmap.nasa.gov. https://humanresearchroadmap.nasa.gov/evidence/reports/SANS.pdf?rnd=0.945882965319735. Accessed October 20, 2020
- ↑ 7.0 7.1 7.2 Mader TH, Gibson CR, Pass AF, et al. Optic disc edema in an astronaut after repeat long-duration space flight. J Neuro-Ophthalmology. 2013;33(3):249-255. doi:10.1097/WNO.0b013e31829b41a6
- ↑ 8.0 8.1 8.2 8.3 8.4 National Aeronautics and Space Administration. Human Adaptation to Space Flight: The Role of Nutrition. https://www.nasa.gov/sites/default/files/human-adaptation-to-spaceflight-the-role-of-nutrition.pdf. Accessed October 20, 2020.
- ↑ 9.0 9.1 Iwasaki K, Levine BD, Zhang R, et al. Human cerebral autoregulation before , during and after spaceflight. J Physiol. 2007;579(Pt 3):799-810. doi:10.1113/jphysiol.2006.119636
- ↑ 10.0 10.1 10.2 Alperin N, Lee SH, Mazda M, et al. Evidence for the importance of extracranial venous flow in patients with idiopathic intracranial hypertension (IIH). In: Acta Neurochirurgica, Supplementum. ; 2005. doi:10.1007/3-211-32318-X_28
- ↑ Hansen H-C, Helmke K. Validation of the optic nerve sheath response to changing cerebrospinal fluid pressure: ultrasound findings during intrathecal infusion tests. J Neurosurg. 2009. doi:10.3171/jns.1997.87.1.0034
- ↑ 12.0 12.1 12.2 Killer HE, Jaggi GP, Flammer J, Miller NR, Huber AR, Mironov A. Cerebrospinal fluid dynamics between the intracranial and the subarachnoid space of the optic nerve. Is it always bidirectional? Brain. 2007. doi:10.1093/brain/awl324
- ↑ Killer HE, Laeng HR, Groscurth P. Lymphatic Capillaries in the Meninges of the Human Optic Nerve. J Neuro-Ophthalmology. 1999;19(4):222???228. doi:10.1097/00041327-199912000-00002
- ↑ Killer HE, Jaggi GP, Flammer J, Miller NR, Huber AR. The optic nerve: A new window into cerebrospinal fluid composition? Brain. 2006;129(4):1027-1030. doi:10.1093/brain/awl045
- ↑ Spaceflight Effects and Molecular Responses in the Mouse Eye: Observations After Shuttle Mission STS-133. Gravitational and Space Research. 2013;1:30-46.
- ↑ Giuseffi V, Wall M, Siegel PZ, Rojas PB. Symptoms and disease associations in idiopathic intracranial hypertension (pseudotumor cerebri): a case-control study. Neurology. 1991.
- ↑ 17.0 17.1 Kramer LA, Sargsyan AE, Hasan KM, Polk JD, Hamilton DR. Orbital and Intracranial Effects of Microgravity: Findings at 3-T MR Imaging. Radiology. 2012;263(3):819-827. doi:10.1148/radiol.12111986
- ↑ Lerner DJ, Chima RS, Patel K, Parmet AJ. Ultrasound guided lumbar puncture and remote guidance for potential in-flight evaluation of VIIP/SANS. Aerosp. Med. Hum. Perform. 2019;90:58–62. doi: 10.3357/AMHP.5170.2019.
- ↑ Bidot S, Bruce BB, Saindane AM, Newman NJ, Biousse V. Asymmetric papilledema in idiopathic intracranial hypertension. J Neuro-Ophthalmology. 2015;35(1):31-36. doi:10.1097/WNO.0000000000000205
- ↑ Ong J, Tarver W, Brunstetter T, et al. Spaceflight associated neuro-ocular syndrome: proposed pathogenesis, terrestrial analogues, and emerging countermeasures. British Journal of Ophthalmology 2023;107:895-900.
- ↑ 21.0 21.1 Taibbi, G. et al. Ocular outcomes comparison between 14- and 70-day head-down-tilt bed rest. Invest. Ophthalmol. Vis. Sci. 57, 495–501 (2016).
- ↑ Kamran, S.A., Hossain, K.F., Ong, J. et al. SANS-CNN: An automated machine learning technique for spaceflight associated neuro-ocular syndrome with astronaut imaging data. npj Microgravity 10, 40 (2024). https://doi.org/10.1038/s41526-024-00364-w
- ↑ 23.0 23.1 Arbeille P, Roumy J. Adaptation of the left heart , cerebral and femoral arteries , and jugular and femoral veins during short- and long-term head-down tilt and spaceflights. 2001:157-168. doi:10.1007/s004210100473
- ↑ Eric J. Power and Kristin Fabre.New Space.ahead of printhttp://doi.org/10.1089/space.2021.0061
- ↑ Xu X, Xu ZM, Liu GY, et al. Effects of head down tilt on intra-ocular pressure, near vision, and visual field and the protection effect of Chinses herbs. Space Medicine & Medical Engineering. 2002(06):419–422.
- ↑ 26.0 26.1 Mader TH, Gibson CR, Otto CA, et al. Persistent Asymmetric Optic Disc Swelling after Long-Duration Space Flight: Implications for Pathogenesis. J Neuro-Ophthalmology. 2017;37(2):133-139. doi:10.1097/WNO.0000000000000467
- ↑ Ong J, Lee AG and Moss HE (2021) Head-Down Tilt Bed Rest Studies as a Terrestrial Analog for Spaceflight Associated Neuro-Ocular Syndrome. Front. Neurol. 12:648958. doi: 10.3389/fneur.2021.648958
- ↑ Arbeille P, Herault S, Fomina G, Roumy J, Alferova I, Gharib C. Influences of thigh cuffs on the cardiovascular system during 7-day head-down bed rest. J Appl Physiol (1985) 1999;87(6):2168–2176.
- ↑ Martin Paez Y, Mudie LI, Subramanian PS. Spaceflight Associated Neuro-Ocular Syndrome (SANS): A Systematic Review and Future Directions. Eye Brain. 2020;12:105-117. Published 2020 Oct 19. doi:10.2147/EB.S234076
- ↑ Hearon CM, Dias KA, Babu G, et al. Effect of Nightly Lower Body Negative Pressure on Choroid Engorgement in a Model of Spaceflight-Associated Neuro-ocular Syndrome: A Randomized Crossover Trial. JAMA Ophthalmol. 2022;140(1):59–65. doi:10.1001/jamaophthalmol.2021.5200
- ↑ Redfern AJ, Lee AG. Spaceflight-Associated Neuro-Ocular Syndrome (SANS). Experts InSight American Academy of Ophthalmology. https://www.aao.org/education/audio/spaceflight-associated-neuro-ocular-syndrome-sans Accessed June 18, 2024.