Retinal Oximetry
All content on Eyewiki is protected by copyright law and the Terms of Service. This content may not be reproduced, copied, or put into any artificial intelligence program, including large language and generative AI models, without permission from the Academy.
Definition
The retina requires a continuous supply of oxygen and is one of the most metabolically active tissues in the human body. In primate models, ischemia greater than 100 minutes was shown to lead to loss of retinal functional and histological measurements[1]. Hypoxia is thought to a major driving force in diseases, such as diabetic retinopathy and retinopathy or prematurity. Therefore, the ability to measure oxygen in the living retina is an important challenge. Retinal oximetry could provide markers of the hypoxic stress of retinal tissue, which could be useful to clinicians monitoring these diseases.
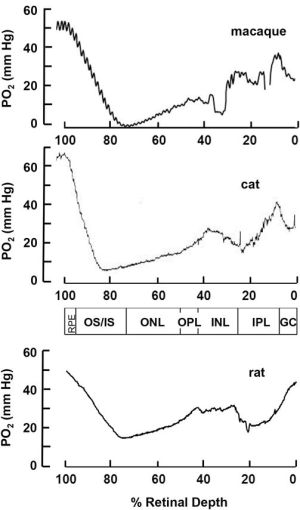
Retinal oximetry broadly refers to technologies that can non-invasively obtain the oxygenation measurements from retinal tissue. Knowledge about retinal oxygenation from the retinal and choroidal circulations has been well-studied from oxygen microelectrode experiments[3]. Microelectrodes were meticulously inserted into retinas of animals, and the measured currents of the microelectrode were proportional to the pressure of O2 (pO2) local to the tip. This technique has provided oxygen profiles (as shown in Figure 1), measurements of pO2 throughout the depth of the retina, which have provided the basis of our understanding of oxygen physiology in the retina.
Imaging technologies have been explored for non-invasive retinal oximetry. Retinal oximetry using imaging generally measures the oxygen saturation of hemoglobin (sO2) from blood within retinal vessels, rather than the pO2 within tissue. Most of the oxygen (~97%) carried within arterioles is bound to hemoglobin, while the remaining percentage is dissolved within plasma. The sO2 is defined as the ratio of the concentration of oxygenated hemoglobin to the total concentration of hemoglobin:
The sO2 is related to the partial pressure of oxygen (pO2) within plasma via a non-linear curve known as the oxyhemoglobin disassociation curve. All methods of oximetry using imaging rely on the differences in extinction coefficients of oxygenated and deoxygenated hemoglobin in the visible spectrum. The most studied method to perform retinal oximetry is based on multi-wavelength fundus photography and the use of the technique has been evolving.[4] Newer approaches for retinal oximetry inpatients include hyperspectral imaging [5], visible-light optical coherence tomography [6], and photoacoustic ophthalmoscopy [7]. This article will focus primarily on the non-invasive measurement technique using fundus photography.
History
The first use of oximetry in a clinical setting was in 1935, when a German physician Karl Matthes developed an ear O2 saturation meter. Early versions of retinal oximetry arose in the 1950s, but advances in digital technology within the past decade have allowed for emergent clinical and research applications. Since 1963 there have been multiple groups who have developed methods to measure retinal sO2 non-invasively. There are currently two commercialized products: the Oxymap Retinal Oximeter (Oxymap ehf, Reykjavik, Iceland; founded in 2002) (Fig. 2,3) and the Vesselmap system (Imedos Systems GmbH, Jena Germany; founded in 1996) (Fig. 3). [8] [9] [10]
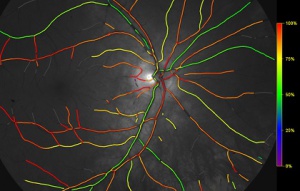
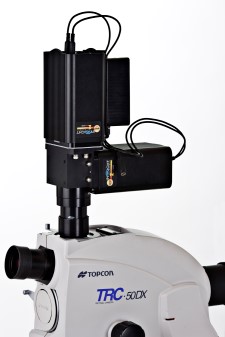
Technique
The foundational principle of retinal oximetry is based on light absorption spectroscopy. Oxygenated and deoxygenated forms of hemoglobin have variations in light absorption across the visible spectrum. Simplistically, arteries and veins have differences in color, which indicates their oxygenation. By measuring the changes in light absorption, calculation of the relative ratio of the two species of hemoglobin can be performed, thus providing a value of the sO2.
In the case of multi-wavelength fundus photography, a fundus camera with an attached image splitter divides the returning light spectrum into distinct multiple, nearly monochromatic wavelength bands that correspond to specific points on the oxygen absorption curve. For example, the OxyMap system uses a splitter that images at two distinct wavelengths: at 600 nm (sensitive to oxyhemoglobin) and 570 nm (not sensitive to oxyhemoglobin). An optical density measurement can be calculated from the measurement of image values within the vessel, I, and brightness values outside the vessel I0, with the equation OD=log(I/I0). The sO2 can be calculated using an equation: SO2= a+k(ODx/ODy) where ODx and ODy are measurements of the OD at wavelengths x and y respectively and a and k are constants.
Limitations
Several factors may influence retinal oximetry measurements using a dual-wavelength method including retinal and choroidal pigmentation, vessel size and thickness, linear blood flow velocity, and retinal nerve fiber layer thickness. These factors have been explored via Monte Carlo simulations as well as with experimental studies, and they should be considered when interpreting results from clinical studies.
Multivariate regression analyses of studies on normative values in healthy subjects has shown no significant associations between retinal oxygen saturation (SO2) and iris pigmentation, ethnicity, gender, and smoking status. Aging, however, is a factor that has been shown in some studies to decrease retinal venous (SvO2) and arterial oxygen saturation (SaO2) and therefore must be accounted for in interpreting and comparing measurements.[8][9][10][11]
Retinal Oximetry in Ocular Diseases
Retinal Vessel Occlusions
Retinal vessel occlusions[12] [13] are blockages of the blood vessels in the retina, often due to atherosclerosis and blood clot formation. There are both central vessel occlusions, near the optic nerve, and branch vessel occlusions, at branches in arterioles or venules. The subsequent blockage of blood flow can lead to blood supply deprivation (ischemia) in areas of the retina. RVOs can be categorized into central RVO (CRVO) or branch RVO (BRVO), depending on the location of the occlusion. Retinal oximetry shows promise in helping differentiate between ischemic and non-ischemic branch RVO (BRVO).
Yang et al. conducted a study to compare arterial and venous oxygen saturation in patients with ischemic and non-ischemic BRVO, as well as healthy individuals. They found that in patients with ischemic BRVO, the venous oxygen saturation in the affected vessels was decreased, while the arterial saturation was increased. Conversely, in non-ischemic BRVO, the mean venous oxygen saturation was lower in the affected vein [14]. In another study by Hardarson et al. [15], venous saturation was found to be more variable . Hypoxia was seen in some eyes, but not in others, which was hypothesized to be due to the variability in disease severity and degree of occlusion.
In central RVO, a larger study linked venous oxygen saturation and arteriovenous difference to a retinal ischemic index measured by ultra-widefield FA [16]. To calculate the retinal ischemic index on FA images, area of capillary nonperfusion seen in the arteriovenous phase image were traced and divided by the total RVO area. The study raised the potential of monitoring the ischemic status of the retina with retinal oximetry.
Diabetic Retinopathy
Diabetic retinopathy[13][17] [18] is a disease caused by damage to the retinal capillaries as a result of chronic hyperglycemia. Similar to retinal vascular occlusions, hypoxia is a central feature of the disease process and pathophysiology. Retinal oximetry has been supportive of theories suggesting blood shunting and bypassing of nonperfused areas occurs in diabetic retinopathy, which leads to increased oxygenation in retinal venules.
Retinal oximetry analysis indicates that patients with retinopathy in general have higher venous oxygen saturation levels than healthy subjects. This seemingly paradoxical oximetry finding is likely due to poor oxygen distribution, increased total blood flow, and/or decreased oxygen consumption[19]. The relative increase in venous oxygenation has been shown to correlate with diabetic retinopathy severity[20][21]. In addition, following laser photocoagulation treatment, the arteriovenous saturations indicate improved distribution of oxygen delivery[22]. As such, retinal oximetry has promising clinical applications for the diagnosis and management of the disease. (Fig 4A & B)
Multiple studies using different other types of oximetry systems have also confirmed higher venous oxygenation in diabetic retinopathy. For example, a study utilizing a scanning laser ophthalmoscope combined with Oxymap software found increased venous saturation in non-proliferative diabetic retinopathy and decreased arterial diameter along with increased venous saturation in proliferative diabetic retinopathy [23]. Another study employed hyperspectral computed tomographic imaging spectroscopy to measure retinal vascular oxygen content, showing lower arteriovenous difference in proliferative diabetic retinopathy patients compared to healthy controls [24].
Glaucoma
Glaucoma[13][25] [26] is a chronic optic neuropathy with progressive injury to optic nerve axons and subsequent atrophy of retinal nerve fiber layer and ganglion cells. This atrophy is expected to reduce oxygen consumption in the inner retina. Consequently, venous oxygen saturation and arteriovenous saturation differences can offer insight into glaucomatous disease progression and provide new modalities of monitoring patients. Research also suggests that retinal vascular dysregulation might be part of the primary pathophysiology in certain types of glaucoma[19]. In addition to aiding in diagnosis and monitoring glaucoma, retinal oximetry might therefore offer new insight into a incompletely understood disease entity.
A 2014 study using the Oxymap system investigated changes in retinal oxygen saturation in glaucoma patients [27]. It found a positive correlation between glaucomatous damage (assessed using visual field defects and retinal nerve fiber layer changes) and oxygen saturation in retinal venules. Conversely, a negative correlation was observed between glaucomatous damage and arteriovenous difference in oxygen saturation.
Additional oximetry studies have shown a mild difference in oxygenation pre- and post-glaucoma treatment, which correlates with intraocular pressure changes[28].
Inherited Retinal Diseases
Studies of retinal oximetry in adults and children with inherited retinal disease have demonstrated reproducible and significant differences in oxygen consumption. The oxygen saturation is increased in patients with IRDs, particular rod-cone dystrophy, due to decreased oxygen consumption. The increased oxygen saturation is hypothesized to contribute to photoreceptor and retinal degeneration through the accumulation of oxygen free radicals. Additionally, the changes in oxygen saturation values have been shown to correlate with structural and functional changes in IRDs as well. As additional investigation is performed, retinal oximetry might emerge as a biomarker to detect progression or to monitor therapeutic effect of future treatments. [29][30][31]
Emerging Applications
In addition to characterizing other ischemic retinal diseases such as age-related macular degeneration and retinitis pigmentosa, retinal oximetry can exist as a potential biomarker for systemic diseases. Investigation into its utility for cardiopulmonary disease states and as a surrogate for systemic oxygenation show early promise, as do studies proposing hypotheses that retinal vascular changes might relate to Alzheimer's disease in the central nervous system. The technology and areas of application continue to evolve.
Additional Resources
References
- ↑ Hayreh SS, Zimmerman MB, Kimura A, Sanon A. Central retinal artery occlusion.: Retinal survival time. Experimental eye research. 2004;78(3):723-36.
- ↑ Linsenmeier RA, Zhang HF. Retinal oxygen: from animals to humans. Prog Retin Eye Res. 2017 May;58:115-151. doi: 10.1016/j.preteyeres.2017.01.003. Epub 2017 Jan 18. PMID: 28109737; PMCID: PMC5441959.
- ↑ Linsenmeier RA, Zhang HF. Retinal oxygen: from animals to humans. Progress in retinal and eye research. 2017;58:115-51.
- ↑ Garg AK, Knight D, Lando L, Chao DL. Advances in retinal oximetry. Transl Vis Sci Technol 2021;105
- ↑ Patel SR, Flanagan JG, Shahidi AM, Sylvestre JP, Hudson C. A prototype hyperspectral system with a tunable laser source for retinal vessel imaging. Invest Ophthalmol Vis Sci. 2013;54(8):5163-8.
- ↑ Shu X, Beckmann L, Zhang HF. Visible-light optical coherence tomography: a review. Journal of biomedical optics. 2017;22(12):121707-.
- ↑ Song W, Wei Q, Liu W, Liu T, Yi J, Sheibani N, et al. A combined method to quantify the retinal metabolic rate of oxygen using photoacoustic ophthalmoscopy and optical coherence tomography. Scientific reports. 2014;4(1):6525.
- ↑ 8.0 8.1 Beach JM, Schwenzer KJ, Srinivas S, Kim D, Tiedeman JS. Oximetry of retinal vessels by dual-wavelength imaging: calibration and influence of pigmentation. J Appl Physiol. 1999;86:748–758.
- ↑ 9.0 9.1 Hardarson SH. Retinal oximetry. Acta Ophthalmologica. 2012;91: 489–490.
- ↑ 10.0 10.1 Hardarson SH, Harris A, Karlsson RA, et al. Automatic retinal oximetry. Invest Ophthalmol Vis Sci. 2006; 47(11):5011-5016.
- ↑ Jani PD, Mwanza JC, Billow KB, et al. Normative values and predictors of retinal oxygen saturation. Retina. 34(2):394-401, 2014
- ↑ Hardarson SH, and E Stefánsson. "Oxygen saturation in central retinal vein occlusion." American journal of ophthalmology 150.6 (2010): 871-875.
- ↑ 13.0 13.1 13.2 Boeckaert J., E. Vandewalle, and Ingeborg Stalmans. "Oximetry: recent insights into retinal vasopathies and glaucoma." Bull Soc Belge Ophtalmol 319 (2012): 75-83.
- ↑ Yang JY, You B, Wang Q, Chan SY, Jonas JB, Wei WB. Retinal vessel oxygen saturation in healthy subjects and early branch retinal vein occlusion. Int J Ophthalmol. 2017;10(2):267-70.
- ↑ Hardarson SH, Stefánsson E. Oxygen saturation in branch retinal vein occlusion. Acta Ophthalmol. 2012;90(5):466-70.
- ↑ Šínová I, Řehák J, Nekolová J, Jirásková N, Haluzová P, Řeháková T, et al. Correlation Between Ischemic Index of Retinal Vein Occlusion and Oxygen Saturation in Retinal Vessels. Am J Ophthalmol. 2018;188:74-80.
- ↑ Hammer M, Vilser W, Riemer T, et al. Diabetic patients with retinopathy show increased retinal venous oxygen saturation. Graefes Arch Clin Exp Ophthalmol. 2009;247:1025-1030.
- ↑ Khoobehi B, Firn K, Thompson H, Reinoso M, Beach J. Retinal arterial and venous oxygen saturation is altered in diabetic patients. Invest Ophthalmol Vis Sci. 2013;54:7103–7106.
- ↑ 19.0 19.1 Stefansson E et al. Retinal oximetry: Metabolic imaging for diseases of the retina and brain, Progress in Retinal and Eye Research, Volume 70, 2019, Pages 1-22,
- ↑ C.M. Jorgensen, S.H. Hardarson, T. Bek. The oxygen saturation in retinal vessels from diabetic patients depends on the severity and type of vision-threatening retinopathy. Acta Ophthalmol., 92 (2014), pp. 34-39
- ↑ B. Khoobehi, K. Firn, H. Thompson, M. Reinoso, J. Beach. Retinal arterial and venous oxygen saturation is altered in diabetic patient. Invest Ophthalmol Vis Sci, 54 (2013), pp. 7103-7106
- ↑ C. Jorgensen, T. Bek. Increasing oxygen saturation in larger retinal vessels after photocoagulation for diabetic retinopathy. Invest Ophthalmol Vis Sci, 55 (2014), pp. 5365-5369
- ↑ Blair NP, Wanek J, Felder AE, Joslin CE, Kresovich JK, Lim JI, et al. Retinal Oximetry and Vessel Diameter Measurements With a Commercially Available Scanning Laser Ophthalmoscope in Diabetic Retinopathy. Invest Ophthalmol Vis Sci. 2017;58(12):5556-63.
- ↑ Kashani AH, Lopez Jaime GR, Saati S, Martin G, Varma R, Humayun MS. Noninvasive assessment of retinal vascular oxygen content among normal and diabetic human subjects: a study using hyperspectral computed tomographic imaging spectroscopy. Retina. 2014;34(9):1854-60.
- ↑ Olafsdottir Olof Birna, et al. "Retinal oximetry in primary open-angle glaucoma."Investigative ophthalmology & visual science 52.9 (2011): 6409-6413.
- ↑ Osborne N. N., et al. "Neuroprotection in relation to retinal ischemia and relevance to glaucoma." Survey of ophthalmology 43 (1999): S102-S128.
- ↑ Vandewalle E, Abegão Pinto L, Olafsdottir OB, De Clerck E, Stalmans P, Van Calster J, et al. Oximetry in glaucoma: correlation of metabolic change with structural and functional damage. Acta Ophthalmol. 2014;92(2):105-10.
- ↑ S. Du, X. Gao, X. Zhang, J. Wang, W. Huang, M. Zhou, W. Wang, X. Li, Y. Zhang, D.S. LamChanges in retinal oxygen saturation, choroidal thickness. , and retinal nerve fibre layer. Can. J. Ophthalmol., 50 (2015), pp. 159-165
- ↑ Bojinova, R.I.; Türksever, C.; Schötzau, A.; Valmaggia, C.; Schorderet, D.F.; Todorova, M.G. Reduced metabolic function and structural alterations in inherited retinal dystrophies: Investigating the effect of peripapillary vessel oxygen saturation and vascular diameter on the retinal nerve fibre layer thickness. Acta Ophthalmol. 2017, 95, 252–261.
- ↑ Della-Volpe, W.M.; Scholl, H.P.N.; Valmaggia, C.; Todorova, M.G. Retinal vessel oximetry in children with inherited retinal diseases. Acta Ophthalmol. 2020
- ↑ Türksever C, López Torres LT, Valmaggia C, Todorova MG. Retinal Oxygenation in Inherited Diseases of the Retina. Genes (Basel). 2021 Feb 14;12(2):272. doi: 10.3390/genes12020272. PMID: 33672973; PMCID: PMC7918478.