Neurodegeneration in Diabetic Retinopathy
All content on Eyewiki is protected by copyright law and the Terms of Service. This content may not be reproduced, copied, or put into any artificial intelligence program, including large language and generative AI models, without permission from the Academy.
Neurodegeneration and neuroprotection strategies have recently become a focus of many researchers within the field of retina. Despite our recent advancements in treating retinal vascular diseases, it is believed that our current treatments are targeting advanced stages of these diseases with mechanisms that do not address the underlying changes or root causes. It has long been believed that angiogenesis and microvascular changes are not the only sources of retinal damage but in fact late presentations, especially in diabetic retinopathy[1].
Neurodegeneration is now thought to play a major role very early in diabetic retinopathy. Research has shown that neurodegeneration occurs in very early stages of the disease. Neurodegeneration has been shown to be present in diabetic human retinas on structural, functional, and molecular levels even in the absence of clinically visible microvascular abnormalities[2][3][4][5]. These studies revealed down regulation of neurotrophic factors such as somatostatin, corticostatin, and pigment epithelium-derived factors as well as thinning of the inner nuclear layer on OCT. Not only is neurodegeneration thought to occur prior to microvascular disease, it is also thought to contribute to it as has been shown in animal models[6].
Despite the advent of anti-VEGF treatments and their profound effect on the field of retina, clinicians are still limited. Due to our limited understanding and lack of tools to address early retinal damage, clinicians are unable to treat these early changes and treatments are only applied when diseases reach an advanced level with visible and sight threatening circumstances. In addition, our ability to treat macular edema is faced with the fact that many patients are still losing vision or not improving after resolution of the edema due to retinal atrophy or macular ischemia.
Disease Entity
Neurodegeneration refers to the cell death of neuronal cells in the retina. Retinal ganglion cells (RGCs) are often the targets of investigations as their loss has been observed in post-mortem histologic examinations and modern OCT imaging in patients with many ocular diseases including diabetic retinopathy[7][8][4].
In addition to retinal vascular diseases, neurodegeneration has been described in almost every disease that causes retinal damage spanning from glaucoma to neuro-ophthalmological conditions along the ganglion cell body tracts from the retina to the lateral geniculate body.
In diabetic retinopathy, it has always been thought to be a late consequence rather than an early manifestation. It was also expected to be found in areas of the retina where the disease is visibly manifested but what was found was rather a global effect underlying those visible manifestations as we will discuss below.
Neurodegenerative mechanisms
Apoptosis and glial activation represent the two major forms of retinal neurodegeneration. As for glial activation, it serves as a major player in many ocular diseases including ocular infections, autoimmune diseases, ischemia, and hereditary retinopathies. Retinal microglia, representing 5-20% of the overall glial population in the retina, play a major role in neurodegeneration. They have a high capability of proliferation with unique interactions with the surrounding astrocytes and neural cells. When they are activated, they exhibit proliferation, migration, phagocytosis, and secretion of many biological factors. The resulting neuronal damage occurs via phagocytosis, production of toxins, and induction of apoptotic changes mentioned above.
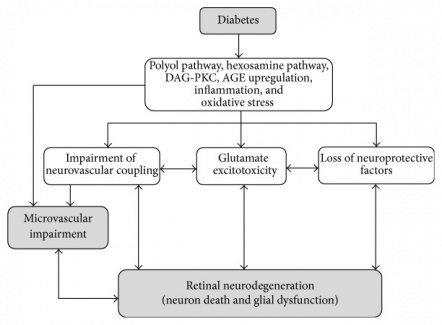
In apoptosis, the nucleus of the cell undergoes condensation and contraction while the cell membranes and organelles remain intact. It is not until the final stages of apoptosis that the cell membranes and organelles become disrupted resulting in cell death.
Understanding the mechanisms of neurodegeneration is important as researchers are hoping to identify key players as targets for treatment and neuroprotection. The following are brief introductions to the mechanisms by which apoptosis and glial activation occur in the retina.
Glutamate excitotoxicity
Excitatory amino acids and their metabolism has been closely studied in many diseases throughout the body including diabetic retinopathy and glaucoma. The level of glutamate in the retina is controlled by a close relationship between neuronal cells and glial cells. Glial cells absorb and metabolise the secreted glutamate from the synaptic terminals to prevent excitotoxic effects and allow the neuronal cells to reabsorb and recycle it in the form of glutamine. Studies show that there is an overexpression of excitatory proteins such as glutamate and NMDA in the retina and vitreous in glaucoma, diabetic retinopathy, and multiple animal models of retinal ischemia. This overexpression is thought to be due to an imbalance in the production and/or metabolism of glutamate. One of the key players in glutamate excitotoxicity is NMDA, one of the ionotropic glutamate receptors. Activation of this receptor leads to calcium influx into the cells followed by the release of caspases leading to apoptosis. In conclusion, toxic levels of glutamate and overexpression of NMDA receptors have been shown to cause damage to retinal neurons [10][11].
Oxidative stress
Reactive oxygen species (ROS) such as nitric oxide (NO) play a significant role in the physiological processes throughout the body including neurotransmission, vasodilation, and host cell defenses[12][13]. However, it has been demonstrated that the release of NO from retinal glial cells induces apoptosis of neuronal cells. This release of NO is thought to be related to overexpression of cytokine-inducible NO synthase (iNOS) in the INL of ischemic retina in animal models of ischemic retinopathies[14][15]. On the other hand, some studies suggest that NO has a neuroprotective effect on the retina and that some neurotrophic factors may execute their effect through alteration of its production or metabolism[16][17].
Other reactive oxygen species are overexpressed in the setting of hypreglycemia as well through activation of the PKC pathway and increase in NADH oxidases. The oxidative stress resulting from ROS and mitochondrial dysfunction mentioned has been observed in diabetic retinas and is thought to induce neuronal apoptosis .
Inflammation
Pro-inflammatory factors have a significant role in many neurodegenerative disorders as well. This includes stroke and Alzhemier’s disease. In the retina, they have also been found to contribute to the pathology of diabetic retinopathy[18]. They are also thought to be one of the causative factor for microvascular changes and capillary loss evident in the early and late stages[19]. Inflammation was shown to exert its effects through pro inflammatory components and advanced ligated end products. These products include advanced glycation endproducts (AGEs) and their receptor (RAGEs)[20]. RAGEs is thought to regulate the responses of retinal glial cells to hyperglycemia and pro-inflammatory molecules such as S100[20]. Other inflammatory factors that play a role here include the transcription factor NF-KB and TGF-B. These factors were shown to be overexpressed in retinal vascular endothelial cells, pericytes, and macrophages[21][22]. These factors thereafter lead to apoptosis of surrounding neuronal cells as well as loss of pericytes and capillary vessels.
Renin-angiotensin system activation
All of the components of the renin angiotensin system (RAS) are reported to exist in the eye[23][24][25]. Angiotensin II in retina functions as a pro-inflammatory factor that activates NF-KB pathway in microvascular endothelial cells[26]. In addition, angiotensin II was shown to have an independent effect on neuronal function in the diabetic retina. Its stimulation of angiotensin II type 1 receptor (AT1R) contributes to retinal dysfunction in the diabetic retina through its effect on synaptophysin, which is a synaptic vesicle protein and a marker of synaptic reduction in neural tissues[27][28].
Overlap Between Angiogenesis and Neurodegeneration
Since neurodegeneration and angiogenesis are simultaneously present in different, it is not a surprise that there is significant overlap between them. Several molecules and factors are thought to have an effect on both pathways. This can be harnessed to our advantage in treating these diseases. For example, insulin-like growth factor 1 (IGF-1) was shown to directly stimulate angiogenesis in animal models and was found to be increased in the vitreous in diabetic retinopathy[29][30]. Furthermore, it was found to be decreased in the serum of premature infants and then later overexpressed as they mature supporting a hypothesis that it may have a role in the early phases of vaso-obliteration and late phases of angiogenesis[31][32]. Simultaneously, IGF-1 is a neurotrophic factor and has been shown to improve neuronal survival. On the other hand, the chronic increase in its levels in transgenic mice resulted in neuronal cell death and neurodegeneration[33]. This highlights the complex nature of these factors and their effects.
Another example is somatostatin, which is decreased in several ischemic retinal diseases. This molecule is thought to decrease angiogenesis through activation of one of its receptors (SST2R) which was shown to decrease VEGF levels[34][35]. In addition, it has been shown to prevent neurodegeneration and cause a decrease in apoptosis and the levels of proapoptotic proteins in the retina[36][37].
Furthermore, some of the key players in the neurodegenerative mechanisms discussed above are shown to have an effect on angiogenic factors. For example, S100B was shown to significantly induce the production of VEGF by retinal glial cells. This effect of S100B failed to mount in RAGE knockdown cells suggesting a central role for the RAGE recptors in this connection between neurodegeneration and angiogenesis[38][39].
Associations
Other retinal vascular diseases
Neurodegeneration is also thought to have a role in other ischemic retinopathies such as retinopathy of prematurity. In animal models of ROP, glial activation and apoptosis were demonstrated as evidence of retinal neurodegeneration[14][28]. Studies on humans with history of ROP revealed decreased rod photoreceptor function independent of laser photocoagulation and disease severity[40][41]. Oxidative stress has been studied in animal models of ROP and targets within that pathway have been shown to reduce neuroglial injury.
Neurodegeneration is also implicated in diseases that involve and acute or subacute ischemic insult. Such conditions include retinal artery occlusions, retinal vein occlusions, and ocular ischemic syndrome. In these ischemic conditions, loss of inner retinal structures is classically evident on OCT imaging with significant loss of ganglion cells. In these conditions, ganglion cell death occurs through two processes: necrosis and apoptosis. Necrotic cell death occurs through swelling of cell bodies and disruption of plasma membranes. On the other hand, cell membranes and organelles remain intact until the final stages in apoptotic cell death through the neurodegenerative materials mentioned above. Multiple experimental ischemic conditions were observed to have apoptotic changes in retinal ganglion cells as well as necrotic changes.
Diagnosis
Diabetic retinopathy is clinically diagnosed when ophthalmoscopic signs are noted on exam. These signs include hemorrhages, microaneurysms, and cotton-wool spots. However, functional defects are known to occur before the onset of these signs. These defects are not apparent to the patient subjectively nor on ophthalmoscopic exam, but have been noted on electrophysiologic and retinal sensory testing.
Regular ophthalmoscopic examinations for patients with diabetes are essential in their care and preventing blindness. However, neurodegenerative effects of diabetes on the retina cannot be seen without specific diagnostic testing designed to examine these changes. The following is a list and brief review of diagnostic procedures used to document and investigate neurodegeneration in clinical practice and research.
Diagnostic procedures
Elecroretinography (ERG)
Multifocal ERG studies performed on diabetic patients revealed evidence of electrophysiologic deficit due to diabetes alone without any evidence of diabetic retinopathy. When correlated with areas of diabetic retinopathy, they were found to be more affected. The deficits reported in these studies were represented by delayed implicit time and abnormal multifocal oscillatory potentials. As for full field ERG studies, the effect of diabetic retinopathy was represented by abnormal oscillatory potentials[42][43].
These changes in mfERG have been used to develop and evaluate a model for prediction of the development of diabetic retinopathy[44]. Over a 3 year period, this model was found to be 88% sensitive and 98% specific[45]. This highlights the consistency and reliability of these findings when clinically apparent diabetic retinopathy is absent.
Contrast Sensitivity
Diabetic patients have been shown to have a significant decrease in contrast sensitivity. The tests used to determine that were steady and pulsed-pedestal paradigms. However, temporal processing speed was not affected in diabetic patients compared to controls[46].
Color Vision Testing
Farnsworth-Munsell 100-hue test has been used to evaluate patients with diabetes mellitus and the test error score was found to be significantly decreased in diabetic patients[47]. In those studies, the patients had normal exams including Ishihara test and fluorescein angiography.
Optical Coherence Tomography
Since OCT allows us to examine the cellular layers of the retina, it was hypothesized that OCT findings may be present prior to any visible diabetic retinopathy. Indeed, studies were able to show thinning of the inner layers of the retina even in the absence of visible diabetic retinopathy[4]. These findings were reported after examining the macular ganglion cell-inner plexiform layer (GCIPL) thickness using the ganglion cell analysis algorithm. This thinning was localized to the papillomacular bundle in the nasal macula, while the temporal macula was not significantly different from normal controls. Furthermore, the thinning was independent of the severity of diabetic retinopathy suggesting that this represents an early change independent of and preceding visible retinopathy.
Visual Field Testing
Retinal sensitivity testing using automated fundus-related perimetry such as microperimeters showed significant decrease in retinal sensitivity in diabetic patients compared to controls in the absence of visible diabetic retinopathy. This decrease in sensitivity was localized to the peripapillary. Foveal and general retinal sensitivity measurements were not not decreased[48].
Management
Neuroprotective Agents
Multiple neurotrophic factors have been found to be decreased in diabetic retinal tissue. The retina synthesizes several neuroprotective molecules with significant levels found in the vitreous of humans. These neuroprotective molecules are the basis of an emerging class of neuroprotective medications that may soon change how we treat diabetic retinopathy. The connection between the neurodegenerative mechanisms and downregulation of the neurotrophic factors is not well understood but it is hypothesized that these neurotrophic factors are the retina’s protective tools against neurodegeneration making them plausible treatment targets for diabetic retinopathy.
Somatostatin (SST) and SST Analogs
SST is a growth hormone inhibiting hormone and one of the major neuroprotective molecules produced by the retina. SST also has antiangiogenic properties and was shown to be decreased in diabetic retinal tissue among other molecules. This molecule is thought to decrease angiogenesis through activation of one of its receptors (SST2R) which was shown to decrease VEGF levels[34][35]. In addition, it has been shown to prevent neurodegeneration and cause a decrease in apoptosis and the levels of proapoptotic proteins in the retina[36][37][49] [50][51]. Topical applications of SST analogs are under investigation for the treatment of diabetic retinopathy and other retinal vascular diseases.
Pigment Epithelium Derived Factor (PEDF)
PEDF is a multifunctional protein that is widely expressed throughout the nervous system and the retina. It has antiangiogenic, antiinflammatory, and neurotrophic properties that inhibit the development and progression of diabetic retinopathy[52][53][54][55]. These properties are attributed to the effect of PEDF on multiple mechanisms including NO expression, RAGE expression, and inhibition of TNF-Alpha induced injury.
Glucagon-like Protein 1 (GLP1)
GLP1 is known to have neuroprotective effects on both the central and peripheral nervous system. The mechanism of action is thought to be protection from glutamate induced apoptosis and AGE induced damage[56][57]. GLP1 receptor was shown to be expressed in human and animal models alike. Furthermore, intravitreal, topical, and systemic GLP1 receptor agonists have been studied in animal models of diabetic retinopathy and were shown to prevent ERG abnormalitis and morphologic features associated with neurodegeneration[58][59].
Future Direction
As our understanding of retinal diseases and their pathologic mechanisms expands, new treatment targets are identified. Now that researchers are identifying neuroprotective targets for diabetic retinopathy among other retinopathies, new treatment modalities may soon emerge. Not only will such treatments expand our treatment options, but they may also preserve retinal tissue leading to less morbidities and improved outcomes especially among patients that are currently challenging and untreatable.
References
- ↑ Antonetti, D. A., Barber, A. J., Bronson, S. K., Freeman, W. M., Gardner, T. W., Jefferson, L. S., … Simpson, I. A. (2006). Diabetic retinopathy: seeing beyond glucose-induced microvascular disease. Diabetes, 55(9), 2401–11. http://doi.org/10.2337/db05-1635
- ↑ Garcia-Ramírez, M., Hernández, C., Villarroel, M., Canals, F., Alonso, M. A., Fortuny, R., … Simó, R. (2009). Interphotoreceptor retinoid-binding protein (IRBP) is downregulated at early stages of diabetic retinopathy. Diabetologia, 52(12), 2633–41.
- ↑ Carrasco, E., Hernández, C., Miralles, A., Huguet, P., Farrés, J., & Simó, R. (2007). Lower somatostatin expression is an early event in diabetic retinopathy and is associated with retinal neurodegeneration. Diabetes Care, 30(11), 2902–8.
- ↑ 4.0 4.1 4.2 Chhablani, J., Sharma, A., Goud, A., Peguda, H. K., Rao, H. L., Begum, V. U., & Barteselli, G. (2015). Neurodegeneration in Type 2 Diabetes: Evidence From Spectral-Domain Optical Coherence Tomography. Investigative Ophthalmology & Visual Science, 56(11), 6333–8.
- ↑ Sohn EH, van Dijk HW, Jiao C, Kok PH, Jeong W, Demirkaya N, Garmager A, Wit F, Kucukevcilioglu M, van Velthoven ME, DeVries JH, Mullins RF, Kuehn MH, Schlingemann RO, Sonka M, Verbraak FD, Abramoff MD. Retinal neurodegeneration may precede microvascular changes characteristic of diabetic retinopathy in diabetes mellitus. Proceedings of the National Academy of Sciences of the United States of America2016;113:E2655-2664.
- ↑ Feng, Y., Wang, Y., Stock, O., Pfister, F., Tanimoto, N., Seeliger, M. W., … Hammes, H.-P. (2009). Vasoregression linked to neuronal damage in the rat with defect of polycystin-2. PloS One, 4(10), e7328.
- ↑ Lorenzi M, Gerhardinger C. Early cellular and molecular changes induced by diabetes in the retina. Diabetologia. 2001;44:791–804.
- ↑ Lieth E, Gardner TW, Barber AJ, Antonetti DA. Penn State Retina Research Group. Retinal neurodegeneration: early pathology in diabetes. Clin Exper Ophthalmol. 2000;28:3–8.
- ↑ Hernández C, Dal Monte M, Simó R, Casini G. Neuroprotection as a Therapeutic Target for Diabetic Retinopathy. Journal of Diabetes Research. 2016;2016:9508541. doi:10.1155/2016/9508541.
- ↑ Ng, Y.-K., Zeng, X.-X., & Ling, E.-A. (2004). Expression of glutamate receptors and calcium-binding proteins in the retina of streptozotocin-induced diabetic rats. Brain Research, 1018(1), 66–72. http://doi.org/10.1016/j.brainres.2004.05.055
- ↑ Pulido, J. E., Pulido, J. S., Erie, J. C., Arroyo, J., Bertram, K., Lu, M.-J., & Shippy, S. A. (2007). A role for excitatory amino acids in diabetic eye disease. Experimental Diabetes Research, 2007, 36150.
- ↑ Christopherson KS, Bredt DS (1997) Nitric oxide in excitable tissues: physiological roles and disease. J Clin Invest 100:2424–2429.
- ↑ MacMicking J, Xie QW, Nathan C (1997) Nitric oxide and macrophage function. Annu Rev Immunol 15:323–350.
- ↑ 14.0 14.1 Sennlaub, F., Courtois, Y., & Goureau, O. (2002). Inducible nitric oxide synthase mediates retinal apoptosis in ischemic proliferative retinopathy. The Journal of Neuroscience : The Official Journal of the Society for Neuroscience, 22(10), 3987–93.
- ↑ Silva, K. C., Rosales, M. A. B., Biswas, S. K., Lopes de Faria, J. B., & Lopes de Faria, J. M. (2009). Diabetic retinal neurodegeneration is associated with mitochondrial oxidative stress and is improved by an angiotensin receptor blocker in a model combining hypertension and diabetes. Diabetes, 58(6), 1382–90.
- ↑ Imanishi T, Kobayashi K, Kuroi A, Mochizuki S, Goto M, Yoshida K, Akasaka T. Effects of angiotensin II on NO bioavailability evaluated using a catheter-type NO sensor. Hypertension 2006;48:1058–1065
- ↑ Lee CI, Liu X, Zweier JL. Regulation of xanthine oxidase by nitric oxide and peroxynitrite. J Biol Chem 2000;275:9369–9376
- ↑ Ramasamy R, Yan SF, Schmidt AM (2005) The RAGE axis and endothelial dysfunction: maladaptive roles in the diabetic vascu-lature and beyond. Trends Cardiovasc Med 15:237– 243
- ↑ Schmidt AM, Hofmann M, Taguchi A, Yan SD, Stern DM (2000) RAGE: a multiligand receptor contributing to the cellular response in diabetic vasculopathy and inflammation. Semin Thromb Hemost 26:485– 493
- ↑ 20.0 20.1 Zong, H., Ward, M., Madden, A., Yong, P. H., Limb, G. A., Curtis, T. M., & Stitt, A. W. (2010). Hyperglycaemia-induced pro-inflammatory responses by retinal Müller glia are regulated by the receptor for advanced glycation end-products (RAGE). Diabetologia, 53(12), 2656–66.
- ↑ Yeh CH, Sturgis L, Haidacher J et al (2001) Requirement for p38 and p44/p42 mitogen-activated protein kinases in RAGE-mediated nuclear factor-kappaB transcriptional activation and cytokine secretion. Diabetes 50:1495– 1504
- ↑ Yan SD, Schmidt AM, Anderson GM, Zhang J, Brett J, Zou YS, Pinsky D, Stern D: Enhanced cellular oxidant stress by the interaction of advanced glycation end products with their receptors/binding proteins. J Biol Chem 269:9889–9897, 1994
- ↑ Danser AH, van den Dorpel MA, Deinum J, Derkx FH, Franken AA, Peperkamp E, de Jong PT, Schalekamp MA: Renin, prorenin, and immu-noreactive renin in vitreous fluid from eyes with and without diabetic retinopathy.J Clin Endocrinol Metab 68:160 –167, 1989
- ↑ Sramek SJ, Wallow IH, Tewksbury DA, Brandt CR, Poulsen GL: An ocular renin-angiotensin system: immunohistochemistry of angiotensinogen. In-vest Ophthalmol Vis Sci 33:1627–1632, 1992
- ↑ Danser AH, Derkx FH, Admiraal PJ, Deinum J, de Jong PT, Schalekamp MA: Angiotensin levels in the eye.Invest Ophthalmol Vis Sci 35:1008 – 1018, 1994 T. KURIHARA AN
- ↑ Nagai N, Izumi-Nagai K, Oike Y, Koto T, Satofuka S, Ozawa Y, Yamashiro K, Inoue M, Tsubota K, Umezawa K, Ishida S: Suppression of diabetes-induced retinal inflammation by blocking the angiotensin II type 1 receptor or its downstream nuclear factor-�B pathway. Invest Ophthalmol Vis Sci 48:4342– 4350, 2007
- ↑ Kurihara, T., Ozawa, Y., Nagai, N., Shinoda, K., Noda, K., Imamura, Y., … Ishida, S. (2008). Angiotensin II type 1 receptor signaling contributes to synaptophysin degradation and neuronal dysfunction in the diabetic retina. Diabetes, 57(8), 2191–8.
- ↑ 28.0 28.1 Downie, L. E., Pianta, M. J., Vingrys, A. J., Wilkinson-Berka, J. L., & Fletcher, E. L. (2008). AT1 receptor inhibition prevents astrocyte degeneration and restores vascular growth in oxygen-induced retinopathy. Glia, 56(10), 1076–90.
- ↑ Meyer-Schwickerath, R., Pfeiffer, A., Blum, W. F., Freyberger, H., Klein, M., Lösche, C., … Schatz, H. (1993). Vitreous levels of the insulin-like growth factors I and II, and the insulin-like growth factor binding proteins 2 and 3, increase in neovascular eye disease. Studies in nondiabetic and diabetic subjects. The Journal of Clinical Investigation, 92(6), 2620–5.
- ↑ Inokuchi, N., Ikeda, T., Imamura, Y., Sotozono, C., Kinoshita, S., Uchihori, Y., & Nakamura, K. (2001). Vitreous levels of insulin-like growth factor-I in patients with proliferative diabetic retinopathy. Current Eye Research, 23(5), 368–71.
- ↑ Hellstrom, A., Perruzzi, C., Ju, M., Engstrom, E., Hard, A. L., Liu, J. L., … Smith, L. E. (2001). Low IGF-I suppresses VEGF-survival signaling in retinal endothelial cells: direct correlation with clinical retinopathy of prematurity. Proceedings of the National Academy of Sciences of the United States of America, 98(10), 5804–8.
- ↑ Hellström, A., Carlsson, B., Niklasson, A., Segnestam, K., Boguszewski, M., de Lacerda, L., … Laron, Z. (2002). IGF-I is critical for normal vascularization of the human retina. The Journal of Clinical Endocrinology and Metabolism, 87(7), 3413–6.
- ↑ Villacampa, P., Ribera, A., Motas, S., Ramírez, L., García, M., de la Villa, P., … Bosch, F. (2013). Insulin-like growth factor I (IGF-I)-induced chronic gliosis and retinal stress lead to neurodegeneration in a mouse model of retinopathy. The Journal of Biological Chemistry, 288(24), 17631–42.
- ↑ 34.0 34.1 Dal Monte, M., Latina, V., Cupisti, E., & Bagnoli, P. (2012). Protective role of somatostatin receptor 2 against retinal degeneration in response to hypoxia. Naunyn Schmiedebergs Arch Pharmacol, 385(5), 481–494.
- ↑ 35.0 35.1 Dal Monte, M., Ristori, C., Cammalleri, M., & Bagnoli, P. (2009). Effects of somatostatin analogues on retinal angiogenesis in a mouse model of oxygen-induced retinopathy: involvement of the somatostatin receptor subtype 2. Investigative Ophthalmology & Visual Science, 50(8), 3596–3606.
- ↑ 36.0 36.1 Hernández, C., García-Ramírez, M., Corraliza, L., Fernández-Carneado, J., Farrera-Sinfreu, J., Ponsati, B., … Simó, R. (2013). Topical administration of somatostatin prevents retinal neurodegeneration in experimental diabetes. Diabetes, 62(7), 2569–78.
- ↑ 37.0 37.1 Kaya, F. S., Akkoyun, İ., Nihan, A., Reyhan, H., & Dağdevİren, A. (2012). Effect of intravitreal octreotide acetate injection on retinal neovascularization , morphology , and apoptotic cell death in an oxygen-induced retinopathy mouse model, 42, 1519–1527.
- ↑ Crawford TN, Alfaro DV 3rd, Kerrison JB, Jablon EP (2009) Diabetic retinopathy and angiogenesis. Curr Diabetes Rev 5:8– 13
- ↑ Nakazawa T, Hisatomi T, Nakazawa C et al (2007) Monocyte chemoattractant protein 1 mediates retinal detachment-induced photoreceptor apoptosis. Proc Natl Acad Sci USA 104:2425– 2430 2666Diabetologia (2010) 53:2656–2666
- ↑ Fulton, A. B., Hansen, R. M., Petersen, R. A., & Vanderveen, D. K. (2001). The rod photoreceptors in retinopathy of prematurity: an electroretinographic study. Archives of Ophthalmology (Chicago, Ill. : 1960), 119(4), 499–505.
- ↑ Fulton, A. B., Reynaud, X., Hansen, R. M., Lemere, C. A., Parker, C., & Williams, T. P. (1999). Rod photoreceptors in infant rats with a history of oxygen exposure. Investigative Ophthalmology & Visual Science, 40(1), 168–74.
- ↑ Bearse MA, Jr., Adams AJ, Han Y, et al. A multifocal electroretinogram model predicting the development of diabetic retinopathy. Prog Retinal Eye Res. 2006;25:425–448
- ↑ Fortune B, Schneck ME, Adams AJ. Multifocal electroretinogram delays reveal local retinal dysfunction in early diabetic retinopathy. Invest Ophthalmol Vis Sci. 1999;40:2638–2651.
- ↑ Han Y, Bearse MA, Jr., Schneck ME, Barez S, Jacobsen CH, Adams AJ. Multifocal electroretinogram delays predict sites of subsequent diabetic retinopathy. Invest Ophthalmol Vis Sci. 2004;45:948–954.
- ↑ Ng, Jason S., et al. "Local diabetic retinopathy prediction by multifocal ERG delays over 3 years." Investigative ophthalmology & visual science 49.4 (2008): 1622-1628.
- ↑ Gualtieri M, Bandeira M, Hamer RD, Damico FM, Moura AL, Ventura DF. Contrast sensitivity mediated by inferred magnoand parvocellular pathways in type 2 diabetics with and without nonproliferative retinopathy. Invest Ophthalmol Vis Sci. 2011;52:1151–1155.
- ↑ Hardy KJ, Lipton J, Scase MO, Foster DH, Scarpello JH. Detection of colour vision abnormalities in uncomplicated type 1 diabetic patients with angiographically normal retinas. Br J Ophthalmol. 1992;76:461–464.
- ↑ Verma A, Rani PK, Raman R, et al. Is neuronal dysfunction an early sign of diabetic retinopathy? Microperimetry and spectral domain optical coherence tomography (SD-OCT) study in individuals with diabetes, but no diabetic retinopathy. Eye. 2009;23:1824–1830
- ↑ Kaya, F. S., Akkoyun, İ., Nihan, A., Reyhan, H., & Dağdevİren, A. (2012). Effect of intravitreal octreotide acetate injection on retinal neovascularization , morphology , and apoptotic cell death in an oxygen-induced retinopathy mouse model, 42, 1519–1527.
- ↑ Simó, R., Lecube, A., Sararols, L., García-Arumí, J., Segura, R. M., Casamitjana, R., & Hernández, C. (2002). Deficit of somatostatin-like immunoreactivity in the vitreous fluid of diabetic patients: possible role in the development of proliferative diabetic retinopathy. Diabetes Care, 25(12), 2282–6.
- ↑ Hernández, C., Carrasco, E., Casamitjana, R., Deulofeu, R., García-Arumí, J., & Simó, R. (2005). Somatostatin molecular variants in the vitreous fluid: a comparative study between diabetic patients with proliferative diabetic retinopathy and nondiabetic control subjects. Diabetes Care, 28(8), 1941–7.
- ↑ S. X. Zhang, J. J. Wang, G. Gao, C. Shao, R. Mott, and J. X. Ma, “Pigment epithelium-derived factor (PEDF) is an endogenous antiinflammatory factor,”FASEB Journal ,vol.20,no.2,pp. 323–325, 2006.
- ↑ J. Spranger, M. Osterhoff , M. Reimann et al., “Loss of the antiangiogenic pigment epithelium-derived factor in patients with angiogenic eye disease,”Diabetes , vol. 50, no. 12, pp. 2641–2645, 2001.
- ↑ S. P. Becerra, A. Sagasti, P. Spinella, and V. Notario, “Pigment epithelium-derived factor behaves like a noninhibitory serpin. Neurotrophic activity does not require the serpin reactive loop,”Journal of Biological Chemistry , vol. 270, no. 43, pp. 25992–25999, 1995.
- ↑ T. Yabe, D. Wilson, and J. P. Schwartz, “NFκ B Activation Is Required for the Neuroprotective Eff ects of Pigment Epithelium-derived Factor (PEDF) on Cerebellar Granule Neurons,”Journal of Biological Chemistry , vol. 276, no. 46, pp. 43313–43319, 2001
- ↑ Lovshin JA, Huang Q, Seaberg R, Brubaker PL, DruckerDJ. Extrahypothalamic expression of the glucagon-like peptide-2 receptor is coupled to reduction of glutamate-induced cell death in cultured hippocampal cells. Endocrinology 2004;145:3495–3506
- ↑ Chen S, An FM, Yin L, et al. Glucagon-like peptide-1 protects hippocampal neurons against advanced glycation end product-induced tau hyperphosphorylation. Neuroscience 2014;256:137–146
- ↑ Zhang Y, Zhang J, Wang Q, et al. Intravitreal injection of exendin-4 analogue protects retinal cells in early diabetic rats. Invest Ophthalmol Vis Sci 2011;52:278–285
- ↑ Hernández C, Bogdanov P, Corraliza L, García-Ramírez M, Solà-Adell C, Arranz JA, Arroba AI, Valverde AM, Simó R. Topical Administration of GLP-1 Receptor Agonists Prevents Retinal Neurodegeneration in Experimental Diabetes. Diabetes. 2016 Jan;65(1):172-87. doi: 10.2337/db15-0443. PubMed PMID: 26384381.